Abstract
Various vanadates have been investigated to accelerate the development of advanced technologies, especially in the area of electronics. Amorphous FeVO4 was synthesized by the liquid phase precipitation method. This precursor was firstly discharged and then charged as cathode material of lithium second battery, in which the valence of two kinds of metals can be changed. Thermal analysis (TG-DTA), powder X-ray diffraction (XRD) and X-ray photoelectron spectroscopy (XPS) have been used for their characterization. The result revealed the existence of two pairs of redox couples, Fe3+/Fe2+ and V5+/V4+. The electrochemical investigation has shown that at higher potentials amorphous FeVO4 can react with 1, 1.5 and 2 mol Li, respectively.
1. Introduction
Globally, driven by the social and economic progress, the newly sustainable, clean, and high-performance energy storage and conversion devices have been highly required [1]. The civilization has been wrapped by a huge number of smart electronics gadgets, especially built with different types of sensors and communication devices, which need a reliable, portable and durable energy storage device [2]. The main classes of electrochemical energy storages are batteries, fuel cells and electrochemical capacitors etc.
Among a variety of energy storage devices, Lithium ion batteries (LIBs), as a renewable power source, have been considered as one of the most important energy storage devices due to their high energy densities, environmental friendly technology, and long cycle and have occupied vast majority of portable electronics market in the last two decades [3]. To meet the recent demand for minimizing the size of portable electronics and electrifying transportation, it is urgent to develop efficient electrode materials for LIBs having a high reversible lithium (Li) capacity, an excellent rate capability, and cycling stability [4].
In a recent past, acceleration in the development of advanced technologies in the area of electronics, various vanadates have been investigated in many fields of application, which can be used as anode material, luminescence material, photocatalyst for degradation of organic pollutants and so on [5]. Many vanadates materials are vital components from an industrial viewpoint and have been proved to be promising due to their low cost, high chemical stability and excellent electrocatalytic properties [6].
As anode material, when discharged to voltage of lower than 0.2 V, FeVO4 can uptake 7 Li. M. Hayashibara et al. [7] studied the chemical lithiation characteristics of crystalline FeVO4. Either amorphous or crystallized FeVO4 displayed reversible capacities of 900 mAhg-1, however they both displayed poor capacity retention. It is found that at lower potentials crystal structure of FeVO4 collapsed and Fe was evident [8].
In this paper we wanted to prepare an amorphous precursor. The precursor was firstly discharged and then charged as cathode material of lithium second battery, in which the valence of two kinds of metals can be changed. FeVO4 as the cathode precursor has seldom been reported. In the range from 3.6 V to 2.0 V vs. Li+/Li, the initial discharge capacity of crystalline FeVO4 was about 70 mAhg-1 [9]. Crystalline FeVO4 will undergo structural amorphization, so the initially amorphous FeVO4 cathode is expected to overcome the irreversible structural change and have better capacity retention than crystalline FeVO4. On the other hand, if vanadium in (VO4)3- polyanion were electrochemically active, there would be more than one lithium intercalation which was favored for high capacity cathode. M. Sugawaraused amorphous FeVO4 as a positive active material and the discharge capacity of the amorphous FeVO4 maintained over 200 mAhg-1 after 50 cycles [10]. They thought the good cycling behavior of amorphous cathode might be attributed to the isotropic, flexible and low-density structures which suppress the deterioration of the cathode during charge-discharge cycles. Herein we prepared amorphous FeVO4 by the soft chemistry method and investigated the electrochemical properties of the amorphous FeVO4 at higher potentials.
2. Experimental section
2.1. Preparation
The starting materials were ammonium metavanadate (NH4VO3) and iron nitrate (Fe(NO3)3·9H2O). The reaction consisted of mixing the stoichiometric amount of the two solutions and controlling the pH. The precipitate was separated by the centrifugation, washed with water and ethanol and then dried at 50 °C overnight. The precursor precipitate was finally calcined at different temperatures for 15 h to get the samples.
2.2. Characterization
Thermal stability was analysed by TG-DTA experiments using a NETZSCH STA 449C apparatus. The precursor precipitate with weight of 12.171 mg was heated in air at a rate of 5 K/min. The power XRD was performed on a RIGAKU D/max-RB diffractometer using Cu Kɑ radiation. XPS data were obtained using an ESCALab 250 electron spectrometer from Thermo Scientific Corporation with monochromatic 150 W AlKα radiation. The base pressure was about 6.5×10-10 mbar. The binding energies were referenced to the C1s line at 284.8 eV from alkyl or adventious carbon.
2.3. Electrochemical properties
The electrochemical properties of the FeVO4 were evaluated by using lithium metal as the anode. A slurry of active material (70wt%) with acetylene black (10wt%) and polyvinylidene fluoride (20wt%) was coated on Al foil to prepare the cathode. One mole LiPF6 in a 1:1 mixture with ethylene carbonate and dimethyl carbonate was used as the electrolyte.
3. Results and discussion
Fig. 1 shows the TG-DTA curve of the precursor FeVO4·nH2O. Five distinct thermal phenomena appear on the DTA curve. The first endothermic peak is located at 97 °C, corresponding to a 12 % loss of water. There are three exothermic effects appeared at 250 °C, 331 °C and 456 °C. M. Touboul et al. have investigated the DTA curve of FeVO4·3H2O. Their results showed the exothermal effect between 230 °C and 260 °C corresponded to the decomposition of NH4NO3. The exothermic phenomena occurred at 330°C and 456 °C were attributed to a modification of the amorphous phase and the crystallization of the compound, respectively. And an endothermic peak appeared at 605 °C, which was different with M. Touboul’s work.
Fig. 2 shows the XRD pattern of the compounds annealed at different temperature. The compounds obtained at 50 °C and 300 °C were both amorphous. The compound annealed at 700 °C was crystalline and was identified as the triclinic FeVO4 (JCPDS 38-1372). We studied the electrochemical performance of the amorphous FeVO4 obtained at 300 °C as cathode precursor.
The voltage-capacity curves and cycle behavior of the amorphous FeVO4 were shown in Fig. 3. As a cathode precursor the amorphous FeVO4 were first discharged and then charged to 4.2 V. By controlling the depth of discharge (DOD), the lithium uptake was calculated by the discharge capacity. The discharge capacity were settled as 157, 235 and 314 mAhg-1, corresponding to 1, 1.5, 2 lithium uptake, respectively. The first two charge-discharge curves at the current of 10 mAg-1 were given in Fig. 3(a), (b) and (c). The slopes of three charge and discharge profiles were slightly steep. And their medium voltage was about 2.7 V, 2.3 V and 2.0 V. The cycle behaviors of the amorphous FeVO4 were shown in Fig. 3(d), (e) and (f). So the amorphous FeVO4 can react reversibly with 1, 1.5, 2 lithium ions, resulting in capacities as large as 157 mAhg-1, 235 mAhg-1 and 314 mAhg-1, respectively. Although their medium voltage was all below 3 V, the energy density of the amorphous FeVO4 reached about 424, 540 and 628 Whkg-1, respectively. So the amorphous FeVO4 could be considered as a potential cathode precursor for lithium second battery.
Fig. 1TG and DTA curves of the precursor FeVO4·nH2O
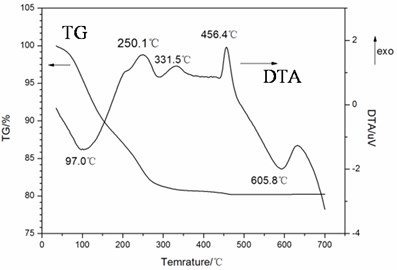
Fig. 2The XRD pattern of the samples annealed at different temperature
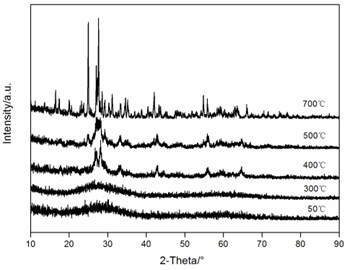
Fig. 3Voltage-capacity profiles and cycle behavior of amorphous FeVO4
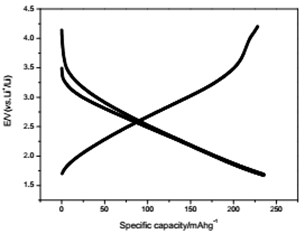
a) Charge/discharge curves for capacity-controlled discharge with 1 lithium embedded
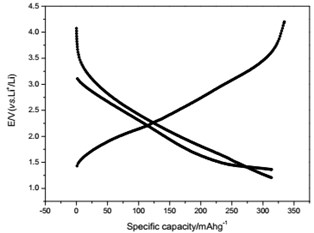
b) Charge/discharge curves for capacity-controlled discharge with 1.5 lithium embedded
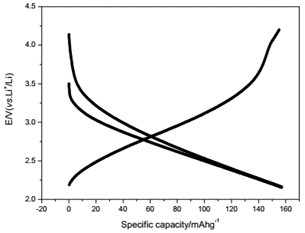
c) Charge/discharge curves for capacity-controlled discharge with 2 lithium embedded
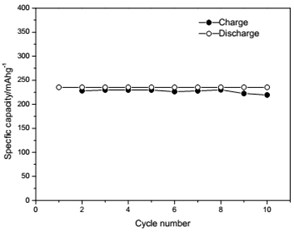
d) Cycling properties of 1 lithium embedded controlled discharge
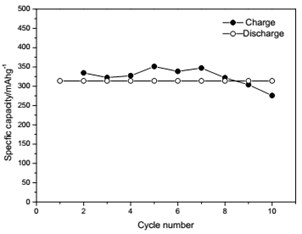
e) Cycling properties of 1.5 lithium embedded controlled discharge
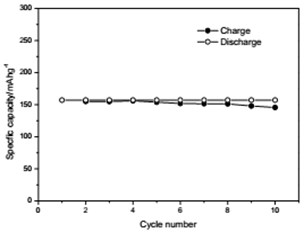
f) Cycling properties of 2 lithium embedded controlled discharge
The cyclic voltammetry (CV) curve of amorphous FeVO4/Li cell in the range of 1.0-4.0 V at a rate of 0.25 mV/s was shown in Fig. 4. During the first cathodic scan (lithium intercalation) the voltammogram showed a broad peak around 2.6 V and an intense peak at 1.1 V. In the first anodic scan (lithium deintercalation) there were two broad peaks around 2.2 V and 3.2 V. In the 10th cycle, the two oxidation peaks (around 2.7 V, 3.4 V) and reduction peaks (around 2.0 V, 3.0 V) became lower and broaden. So the first cycle maybe an activated process. The CV featured two pairs of oxidation-reduction peaks, which was probably associated with both redox couples, Fe3+/Fex (x=+2, 0) and V5+/Vy(y=+4, +3).
Fig. 4Cyclic voltammetry of amorphous FeVO4/Li cell for the 1st and 10th cycle (0.25 mV/s)
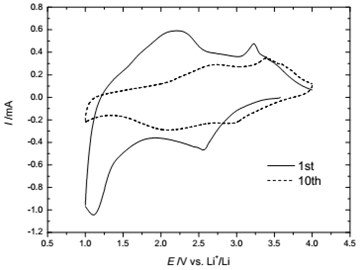
To provide evidence supporting our hypothesis about two redox couples, Fe3+/Fex (x = +2, 0) and V5+/Vy(y=+4,+3), the XPS investigation was performed. Fig. 5 represented the voltage-composition profiles of amorphous FeVO4/Li cell in the first cycle. Different LixFeVO4 (x = 0, 1, 2) compositions were labeled from A to E. The start point of the cell is A. Firstly the discharge process was A→B→C. And then the charge process was C→D→E. Fig. 6 showed the Fe 2p and V 2p XPS spectra of different LixFeVO4 compositions during the first discharge-charge cycle. The binding energy fitting parameters and valence of Fe and V were reported in Table 1. The Fe 2p3/2 peak at 711.5eV and V 2p3/2 peak at 517.5eV could be assigned to Fe3+ and V5+ in the as-prepared amorphous FeVO4 precursor (sample A). When discharged to uptake 1 Li (sample B), the binding energy of the Fe 2p3/2 peak could be fitted to those of Fe3+ (711.2eV) and Fe0 (705.7eV), the binding energy of the V 2p3/2 peak fitting to those of V5+ (517.1eV). After full discharge to uptake 2 Li (sample C) the valence of Fe and V was 0 (705.7eV) and +4 (516.1eV), respectively. Sample D had too small amount of Fe to have evident Fe 2p peaks and the peak of V 2p3/2 could be fitted to those of V5+ (517.1eV) and V4+ (516.4eV). After full charge to remove 2 Li (sample E), the peak of Fe 2p3/2 changed to the position of Fe2+ (710.9eV, 715.2eV). And the peak of V 2p3/2 shifted to higher binding energy and could be fitted to those of V5+ (517.1eV). The result was consistent with the CV feature and demonstrated the existence of both redox couples, Fe3+/Fex (x = +2, 0) and V5+/Vy(y = +4), and the details are shown in Table 1.
Table 1The binding energy fitting parameters and valence of different sample
Sample | Fe 2p3/2 /eV | Valence of Fe | V 2p3/2 /eV | Valence of V |
A | 711.5 | +3 | 517.5 | +5 |
B | 711.2, 705.7 | +3,0 | 517.1 | +5 |
C | 705.7 | 0 | 516.1 | +4 |
D | – | – | 517.1, 516.4 | +5, +4 |
E | 710.9, 715.2 | +2 | 517.1 | +5 |
Fig. 5Discharge-charge profile of amorphous FeVO4/Li cell. A: discharge initially, B: discharge until 1 lithium is embed, C: discharge until 2 lithium are embed, D: charges until 1 lithium is taken off, E: charges until 2 lithium are taken off
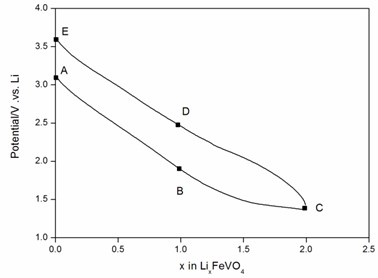
Fig. 6The XPS spectra of different LixFeVO4 compositions: a) Fe 2p and b) V 2p
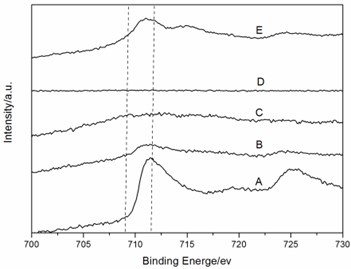
a)
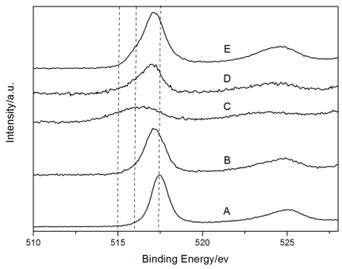
b)
4. Conclusions
In this paper we focus on the reverse lithium embedding of ferrovanadate and try to verify whether double valence transformation occurs during the reaction of ferrovanadate with lithium. The chemical composition of the amorphous form was first confirmed by precipitating the amorphous form by the liquid phase method. Charge and discharge experiments demonstrated that amorphous ferrovanadate can react with 1, 1.5 and 2 lithium at higher potentials with discharge capacities of 157, 235 and 314 mAh g-1, respectively, and can remain largely stable after 10 weeks of cycling. The capacity and energy density of the amorphous FeVO4/Li cell can reach 314 mAhg-1 and 618 WhKg-1, respectively. The existence of two pairs of redox couples in amorphous FeVO4, Fe3+/Fex (x = +2, 0) and V5+/Vy(y = +4), was demonstrated by CV and XPS measurement. Therefore, the amorphous FeVO4 could be considered as potential cathode precursor for lithium second battery.
References
-
L. Fang et al., “Facile synthesis of hierarchical porous carbon nanorods for supercapacitors application,” Applied Surface Science, Elsevier BV, 2019.
-
Q. Wang et al., “Research progress on vanadium-based cathode materials for sodium ion batteries,” Journal of Materials Chemistry A, Vol. 6, No. 19, pp. 8815–8838, 2018, https://doi.org/10.1039/c8ta01627e
-
Z. Wei, L. Wang, M. Zhuo, W. Ni, H. Wang, and J. Ma, “Layered tin sulfide and selenide anode materials for Li- and Na-ion batteries,” Journal of Materials Chemistry A, Vol. 6, No. 26, pp. 12185–12214, 2018, https://doi.org/10.1039/c8ta02695e
-
Q. Li, Y. Chen, J. He, F. Fu, J. Lin, and W. Zhang, “Three-dimensional VS4/graphene hierarchical architecture as high-capacity anode for lithium-ion batteries,” Journal of Alloys and Compounds, Vol. 685, pp. 294–299, Nov. 2016, https://doi.org/10.1016/j.jallcom.2016.05.293
-
W. Guo, X. Li, J. Xu, H. K. Liu, J. Ma, and S. X. Dou, “Growth of highly nitrogen-doped amorphous carbon for lithium-ion battery anode,” Electrochimica Acta, Vol. 188, pp. 414–420, Jan. 2016, https://doi.org/10.1016/j.electacta.2015.12.045
-
S. J. Hu, J. Yang, and X. H. Liao, “Highly efficient degradation of methylene blue on microwave synthesized fevo4 nanoparticles photocatalysts under visible-light irradiation,” Applied Mechanics and Materials, Vol. 372, pp. 153–157, Aug. 2013, https://doi.org/10.4028/www.scientific.net/amm.372.153
-
M. Yeganeh Shad et al., “Wet-chemical synthesis and electrochemical properties of Ce-doped FeVO4 for use as new anode material in Li-ion batteries,” Journal of Inorganic and Organometallic Polymers and Materials, Vol. 23, No. 6, pp. 1226–1232, Nov. 2013, https://doi.org/10.1007/s10904-013-9909-7
-
Y. V. Kaneti, Z. Zhang, J. Yue, X. Jiang, and A. Yu, “Porous FeVO4 nanorods: synthesis, characterization, and gas-sensing properties toward volatile organic compounds,” Journal of Nanoparticle Research, Vol. 15, No. 9, p. 1948, Sep. 2013, https://doi.org/10.1007/s11051-013-1948-z
-
D. Hao Sim et al., “Direct growth of FeVO4 nanosheet arrays on stainless steel foil as high-performance binder-free Li ion battery anode,” RSC Advances, Vol. 2, No. 9, p. 3630, 2012, https://doi.org/10.1039/c2ra20058a
-
S. Patoux and T. J. Richardson, “Lithium insertion chemistry of some iron vanadates,” Electrochemistry Communications, Vol. 9, No. 3, pp. 485–491, Mar. 2007, https://doi.org/10.1016/j.elecom.2006.10.006
About this article
The authors acknowledge the financial support by National test and evaluation platform for advanced materials – Primary center (TC170A5SU/1) and National test and evaluation platform for advanced materials – Nonferrous metals center (TC190H3ZW/2).