Abstract
Investigate whether different results about the physical properties of the same biomaterial frequently found in the literature are due to chewing simulator deficiency. A literature review was performed by searching for data on indicators of test accuracy, reproducibility, maintenance of test parameters during all experiments, and standards in the articles or in the sites of manufacturers. The database searched was CAPES PORTAL, and the keywords used were “bite force” AND “simulator”, “chewing simulator” and “mastication simulator”. Including criteria for the papers are publication filter date of “January 1, 2016”, articles in English, Spanish and Portuguese language were accepted. The first 100 papers that seemed suitable when considering the title and abstract were recovered. Only one simulator used for food property studies had the parameters searched. In the customized simulators for biomaterial properties, only one showed standards, and 8 showed all other parameters searched. All manufactured simulators showed all parameters searched, and only two manufactures did not show standards. Based on the data obtained, the disparate results of experiments with dental materials appeared to be more related to the test conditions than to the testing machines. Knowledge Transfer Statement The findings of the current review suggest that the chewing simulators used in preclinical research are reliable, bringing safety to restorative processes regarding the material. Any difference in preclinical investigations about dental material physical properties is due to test conditions other than chewing simulators.
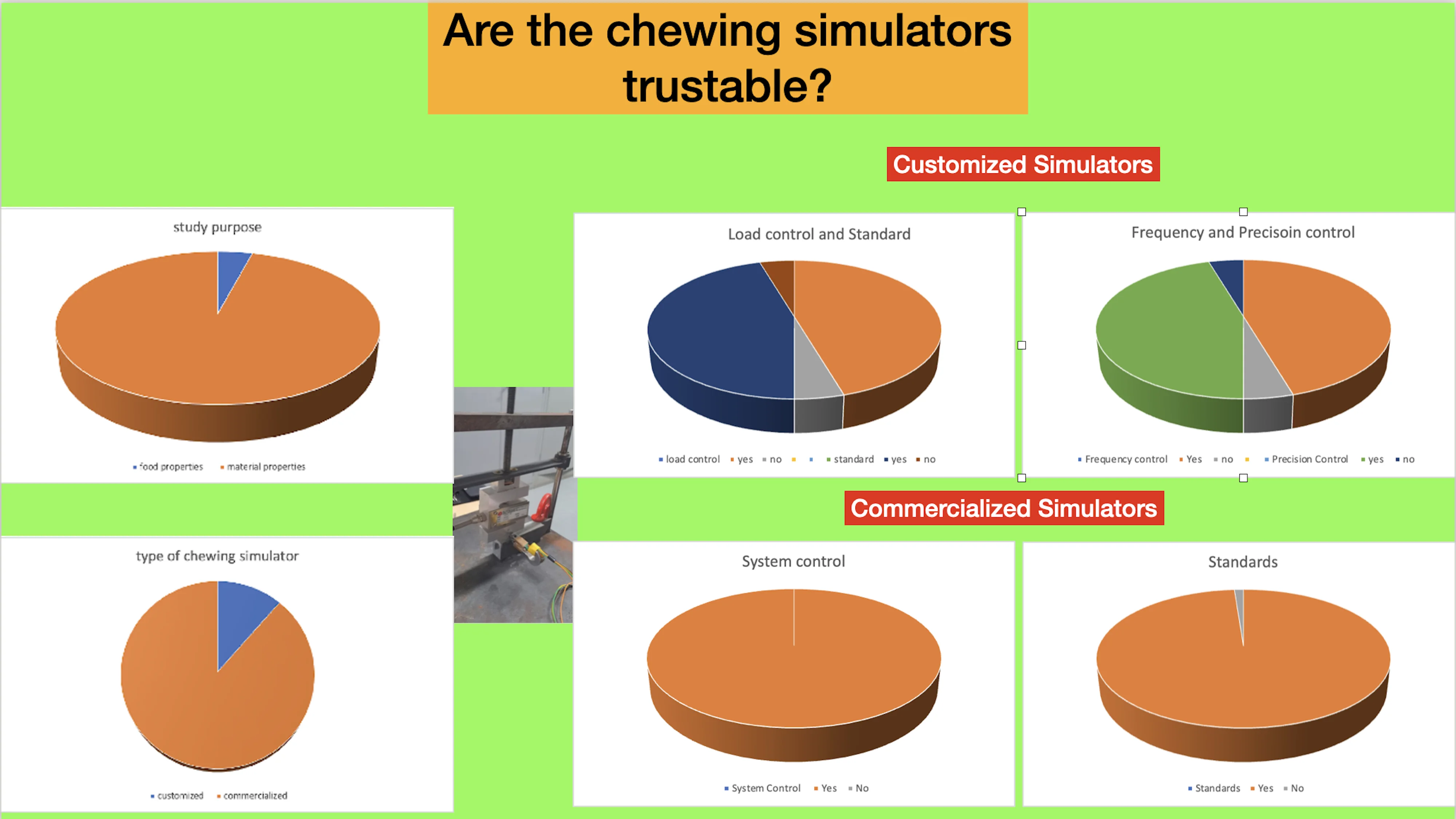
Highlights
- Efficiency of chewing simulators ensures proper physical quality of new release material of JFO.
- Chewing simulators properties are adequate to pre clinical tests of new released dental material.
- If submitted to the correct test, dental material properties are trustable.
1. Introduction
Since the end of the 19th century, there has been concern in dentistry about the progress of science and new technologies for improvement in clinical practice [1]. The number of dental materials and manufacturing methodologies have increased considerably in recent years. Knowledge of human bite force and the physical properties of restorative materials and their biocompatibility is fundamental in the practice of dentistry to perform the required anatomical and functional restoration of the stomatognathic system (SS).
Simulation of the behavior of living tissue is the main goal of applied parameters in the wear test mechanisms carried out in the laboratory environment to preclinical biomaterial tests. Fatigue and abrasion load of biomaterials are important in achieving their mechanical behavior to release them for clinical research. The procedures are interconnected and fundamental for in vitro studies. The basic difference between a two-body and a three-body abrasion wear test is that, in the first one, the grits or hard particles adhere to a surface, and the material is removed from the surface; in the last one, the particles are free to roll and slide down a surface. Both procedures are used to study the wear pattern of a biomaterial. In fatigue tests, the biomaterial is subjected to conditions that produce fluctuating stresses and looks for cracks or complete fractures after a predefined number of fluctuations [2]. That is, the wear test studies the resistance of the biomaterial to abrasion, and the fatigue test studies the resistance of the biomaterial to failures.
In the 19th century, Black [3] measured the bite force to understand the characteristics of the human tooth in relation to the pathologies that affected it and to obtain information to establish the physical properties necessary for a restorative material.
Correct chewing with an ideal bite force is the most important factor in the morphofunctional maintenance of SS and brain health (brain-stomatognathic axis) since chewing stimulates the release of neurotransmitters such as serotonin, dopamine and mineralocorticoids in the central nervous system (CNS). These neurotransmitters contribute to well-being, protect against autonomic cardiac arrhythmias, improve learning conditions and slow brain aging [4-11]. Clearly, inadequate restorative procedures and/or materials can compromise these benefits provided by mastication.
Before testing the material in the mouth, it must undergo a preclinical test to determine its resistance to fatigue, wear, fracture, and effect on antagonist teeth, among other properties. This is why the application of specific loads to this material is necessary to simulate the conditions of the SS by means of a testing machine. Another use of testing machines is to study the effects of chewing on food boluses [12, 13]. There are simulators that serve both purposes such as those made by SD Mechatronik (Mechatronik GMBH, Feldkirchen-Westerham) [14].
Due to the insertion of the CAD/CAM process of obtaining restorations and implants in dentistry and the increasing number of polymers that can be used in this process, testing machines are fundamental, although they are not always used [15].
According to one study [15], some restorative materials have been for sale on the market for a long time without having undergone preclinical tests, especially metal porcelain, thereby placing patients at risk. Furthermore, one study [16] reported that in the last century, none of the wear test machines used in dentistry were included in an international standard. In 2001, standards were established for testing the wear resistance of dental materials in two- and three-body wear machines in accordance with the International Organization for Standardization (ISO) technical specifications [16].
In 2006, a study [17]similar to the present was conducted and, at the time, reported that only MTS simulators (MTS Corp., Minneapolis, MN, USA) were qualified for in vitro wear testing. According to the author, the force exerted by the actuator was controlled and regulated throughout the test only in the MTS simulators. The weak reproducibility of the tests would be the explanation for the great variation of results found at the time.
The load simulators are divided into three major groups: static load simulators, dynamic load simulators, and mixed simulators that can generate static and dynamic loads. Dynamic load simulators provide more data such as tension, bend, durability, high cycle fatigue, low cycle fatigue, compression, creep, fatigue, crack growth, and fracture toughness [18] than static load simulators; however, they have a higher cost and are less accessible to research (fewer job places on the bench) [15].
In this database search, the first simulator found in the literature [19] used a dry skull to determine the force necessary for crushing food during human chewing, with the purpose of using the information for the development of restorative materials. He performed the test by placing the skull upside down, then he attached a bucket to its jaw by means of wires, and placed weights in it (bucket) to determine what pressure was needed for crushing the food.
It is important to emphasize that test conditions may play an important role in the results found in the investigations of biomaterial physical properties. Differences in test conditions, for instance, in the wear mechanism, static or dynamic load, two or three body wear tests, fatigue wear tests [18, 20-23]etc.; thermal cycling [24, 25]; and test environmental conditions [15, 26-28] may significantly affect biomaterial in vitro investigation results, but this will not be considered. Since the ability to grind food is dependent, among other factors, of the quality of the anatomy and functionality of dental restorations and the quality of the material used, the aim of this paper was to review the literature since 2016 to evaluate whether the simulators used in the literature, based on the technical data found in the respective websites and/or in the published studies of customized simulators, are able to maintain test conditions during all experiments, ensuring their accuracy during the tests and the reproducibility of the tests to ensure excellence in occlusal plane restoration when needed.
2. Methodology
The search was conducted in two databases, both accessed on 27/06/2020. with the following keywords: "bite force" AND "simulator", "chewing simulator" and "mastication simulator". In PubMed (https://pubmed.ncbi.nlm.nih.gov/), using the date of publication filter “5 years”. This time interval was chosen based on the PubMed timeline. Searching for biomaterials, there can be an increase in the number of published papers about the subject and a decrease at the end of the period that can be seen in the graph (https://pubmed.ncbi.nlm.nih.gov/?term=(biomaterials)%20AND%20 ((%222015%2F01%2F01%22%5BDate%20%20Publication%5D%20%3A%20%222019%2F01%2F01%22%5BDate%20-%20Publication%5D))&sort=&timeline=expanded). In the PubMed database, 204 articles were found, and in CAPES PORTAL (http://www-periodicos-capes-gov-br.ez27.periodicos.capes.gov.br), 403 articles were found. We opted to use the data from the CAPES PORTAL, a Brazilian government database that allows students enrolled in public federal universities in Brazil to download the papers without cost. Of the 403 articles found in the search, 100 articles were recovered based on the title and abstract. Articles whose titles and abstracts did not seem to be suitable were not recovered. Three articles were excluded due to the methodology applied; the researchers used no testing machine and only finite element method analysis [29-31].
In the other 97 articles, two distinct groups of chewing simulators were found: those manufactured by industries and commercialized (for sale) (from now on, this type will be referred to as commercialized simulators) and those developed by universities, research centers, or research groups (from now on, these will be referred to as customized simulators). In the former group, the technical information and standards were searched on the manufacturer's website. In the customized group, technical information was searched in the paper itself or in another paper if cited. Each study was checked independently by two reviewers. The data obtained were put in an Excel spreadsheet. The data were reviewed by each reviewer independently and then discussed together to avoid bias of wrong and/or missed information.
2.1. Testing machines for food study
Among the studies recovered, 4 [12-14, 32] used the chewing simulator to study the substances released while the food bolus was being chewed. A Cs simulator from SD Mechatronik (Feldkirchen-Westerham, Germany) was used in one of the studies [14] and will be evaluated later when evaluating the simulators for the study of the properties of restorative materials (Fig. 1).
Fig. 1Study purpose. The graphic shows the distribution of studies about food properties and dental material physical properties used in the review
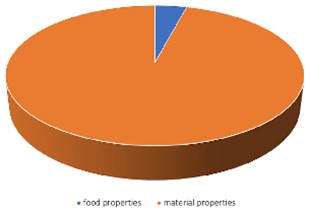
Two studies [12, 13] used the customized simulator developed and described in another paper [33]. This customized simulator consisted of an active cell, where the chewing process was performed, an electronic control box, and a computer to monitor and adjust each parameter. The active cell was composed of a movable jaw and a fixed jaw; both jaws were ring-shaped cylinders fitted with molars made of polyether ether ketone (PEEK) cylinders and a tongue, exerting a force of 30 dekanewtons and a shear force of 35 dekanewtons at an angle corresponding to 1/8 of the tooth. Although we had no access to more technical information, the data described in the studies led us to believe that this customized simulator was efficient and would allow test reproducibility and accuracy (Table 1).
The AM2 chewing simulator used in one study [32]was validated anteriorly [34]. This simulator has two discs, one fixed, corresponding to the maxilla, and one mobile, corresponding to the mandible. The shape of the discs differed from human dental anatomy but was similar to contact surfaces at work as in human chewing. According to the authors, the food bolus resulting from crushing in the simulator was statistically equivalent to the food bolus crushed by human chewing regarding particle size (Table 1).
Table 1Efficiency of chewing simulators for food property studies
Reference | Crushing precision | Reproducibility of the test | Standards |
12 | Yes | Yes | No |
13 | Yes | Yes | No |
14* | Yes | Yes | Yes |
32 | Yes | Yes | No |
* Manufactured by mechatroniks |
2.2. Chewing simulator for testing the physical properties of materials
2.2.1. Customized
The customized simulators were used in 11 of the articles recovered (Fig. 2). The chewing simulator developed by the University of Zurich was used in two studies [35, 36] for fatigue testing. According to the authors, this chewing simulator generated a load of 49 N, and the frequency and number of repetitions could be adjusted. In addition, this simulator allowed a thermal cycling process operating between 5 and 50 degrees Celsius, but no monitoring and controlling devices or systems were reported.
Fig. 2Chewing simulators manufacture. Graphic shows the distribution of customized and commercialized chewing simulators used for dental material property studies.
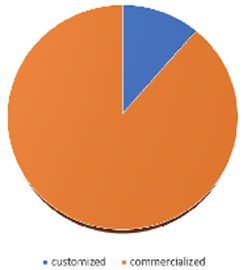
A chewing simulator for in vitro measurement of unidirectional and bidirectional loads was reported in one study [37]. A system for monitoring and controlling the frequency of the load was reported, but no load intensity and precision monitoring and/or controlling systems were reported.
One reported wear simulator consisted of two aluminum discs, one fixed bottom, and one upper rotating at 10 rpm, driven by a Johnson engine with a capacity of 5 kg. According to the authors [38], the contact geometry was representative of the masticatory function. The authors did not report any electronic process monitoring and controlling devices.
A customized simulator developed by the Fourth Military Medical University [39]used spheres as antagonists to investigate the tribological response of dental crowns to cyclic loads. After thermal treatment, the spheres made of stainless steel were similar to the natural enamel. The authors did not describe any mechanism for monitoring and controlling the process to maintain the same conditions during a test and its reproducibility.
The Frankfurt chewing simulator that was designed and built specifically to test implant connections was used in one study [40]. A two-dimensional load could be applied, simulating the chewing forces as much as possible. Two actuators driven by Lorenz force were used for load generation at a 90° angle. The strength and time variables were adjustable. The force produced by the actuators could be generated in arbitrary directions between +90° and –90° in relation to the long axis of the implant and ranged from zero to 300 N. Actuators were controlled by an electronic amplifier, which in turn was controlled by the LabVIEW Graphics Programming System® [41]. From the authors' description, this simulator was a machine that allowed the same conditions to be maintained throughout the test, ensuring reproducibility of the test [40, 41].
A chewing simulator developed by the University of Selçuk (Research Laboratory Centre, Konya, Turkey) was used in a study [42]. The simulation chambers were filled with artificial saliva that was maintained at 37 °C throughout the test period, and occlusal contacts occurred under simulated physiological conditions. The dynamic load was obtained with a pin-on-block design with 0.5 mm glides at a frequency of 1.2 Hz with a constant load of 50 N per 20,000 cycles.
A chewing simulator developed to aid the placement of implants was presented in a study [43]. This simulator had a robotic chewing system with 6 degrees of freedom, with hydraulic actuators that replicated the action of the jaw to replicate the forces occurring in normal chewing. The authors reported a control system to maintain constant hydraulic pressure in the actuators. Test accuracy was guaranteed because the kinematic structure was a redundant robot arm - SCARA (Selective Compliance Assembly Robot Arm). Industrial grade systems have also been developed to conduct monotonic and cyclic tests, which are predominantly uniaxial compression and tensile testers. These systems have also been used for (ISO) 14801 standardization testing.
A bite force simulator composed of pistons supported by a customized impression of the individual's skull obtained by 3D printing was reported [44]. Muscle loads were generated by a proportional pressure controller (PPC5C; MAC Valves, Wixom, MI, USA) operated by LabVIEW software (National Instruments, Austin, TX, USA) by means of an analog voltage controller.
A biaxial mastication simulator produced and developed by the authors was reported in two studies [16, 45]. This simulator exerted a 50 N load on the vertical axis and a horizontal slide of 0.7 mm when the simulator came into touch with the studied specimen. This touch was controlled by magnetic sensors so that after (touching the specimen), the load returned to the beginning, allowing the load to be exerted at the same point, and accurately reported the point of force application; however, the authors did not report any frequency and load controlling systems or devices.
2.2.2. Commercialized simulators
A computer-controlled biaxial chewing simulator [46, 47], a universal fatigue simulator [48], and an artificial aging machine simulating 5 years of use [49-51] were manufactured by Willytec (Feldkirchen-Westerham, Germany). The previous name of SD Mechatronik [15] simulators was used in 6 studies.
The chewing simulator manufactured by EGO (Regensburg, Germany) with Willytec technology was used in 6 studies [52, 57]. This simulator allowed thermal cycling, operating between 5° and 55 °C and testing with vertical and horizontal movements. Apart from citing the Willytec technology, there was no report on the manufacturer’s website about the standards used for the manufacture of simulators, devices or monitoring and control systems [58].
SD Mechatronik chewing simulators (Mechatronik GMBH, Feldkirchen-Westerham, Germany) CS 4.2 have been indicated for determination of the resistance to composite wear, fracture resistance of crowns, bridges and implants, and simulation of bruxism for interocclusal plate testing. According to the manufacturer, these simulators have a high degree of reproducibility of the results because they allow identical kinematic conditions for each species placed in the test chambers. Model CS 4.8 simulates various masticatory movements to test implants, bridges, crowns, composites and jaw models. Model CS 4.8 performs the complete masticatory cycle through linear motion along 2 axes, simulating chewing frequency up to 5 Hz, simulating bruxism for interocclusal plate testing, and can offer real-time wear analysis. The two models provide thermal cycling operating between 5 and 55 degrees Celsius [59] and were used in 35 studies [14,15,60-91], although one of the studies [88] reported the use of CS 4.10, but the model was not found on site. These simulators were all produced with Willytec technology. According to the site, the simulators complied with the following standards: ISO 14801: 2017, ISO 6872: 2019, ISO 10477: 2017 and ISO/TS 14569 [59].
Instron (INSTRON, Norwood, MA, USA) manufactures several universal simulators that have proven to be efficient in preclinical tests, each simulator related to the type of material tested. In this review, the following models have been used in the studies: E3000 [86], 4411 [62] for static load testing, 4465 [93], 8501 [94], 8871 [95], 5848 MicroTester [96] that tested laminates and bond strength, the universal test machine [97], EMIC DL 2000 [98], 3344 [99] for fracture testing. The testing machine from Instron (Norwood, MA, USA) can be used for determining the strength of materials in the following types of tests: tensile, compression, bending, shear, pullout, delamination, adhesion, inlay, etc., that is, all classes of destructive mechanical testing. This equipment, which performs rupture tests at high temperatures, is supplied with the Bluehill testing software. Instron test machines comply with ISO 6892-2, EN 10002-5, and ASTM E21 standards [100].
Zwick/Roell (Ulm, Germany) offers several dynamic simulators, among which the choice depends on the properties of the material to be tested and the desired load. All models listed below were used for preclinical testing. According to the manufacturer, all models have test control II software that allows a very precise positioning of the tested part, measurement and test reproducibility. The Z050 model is indicated for testing fatigue, indentation hardness, and compression stress. All tests are performed in 3 phases: in the first, the thickness and hardness of the indentation are measured; in the second, a fatigue load between 8,000 and 150,000 cycles is given, depending on the material tested; and in the next stage, the reduction in thickness and load for loss of interdentation is tested. The Z 005 model offers compression testing up to 5 kN and thermal testing; Model Z 1445 offers a pressure of up to 10 kN indicated for fracture testing in flexible polymers. The Z010 model offers a pressure of up to 10 kN, and ZwickiLine 2.5 kN offers a pressure of up to 2.5 kN [18]. These simulators were used in 15 recovered articles [101-115]. Zwick/Roell manufacturing test machines comply with ISO 9001 and 14001 standards [18].
The Robota Company (Alexandria, Egypt) chewing simulator used in one study [116]is equipped with a servo-oriented steering system, motors for horizontal and vertical movements, adjustment of the distance of movement integrated with thermal cycling and adjustment of the level of the active tip for accurate positioning [117].
One study [118] used the ANSYS program for Finite Element Method study and Shimadzu’s (Kyoto, Japan) AG-10kNXplus universal test machine for static and dynamic load testing. The device has trapezium X processing software that ensures reproducibility in all tests regarding the intensity and vector of load applications and complies with ISO 6892: 2016 (JIS Z 2241:2011) standards [119].
To test the fatigue of direct intracoronal restorations, two studies [120, 121] used the Biocycle V2 pneumatic chewing simulator from Biopdi (São Carlos, Brazil). This equipment performs mechanical fatigue tests that closely simulate the conditions found in the human mouth and improves the in vitro study research methods that simulate human mastication. Compression or impact tests can be performed with forces that can range between 0 and 200 kgf. Because it is powered by compressed air, this simulator can reach frequencies of up to 5 Hz without losing efficiency and up to 10 Hz in specific cases. This simulator also has a sliding system for tests that best simulate masticatory movement, such as bruxism tests and is provided with software for making adjustments, configurations, acquisition, analysis, and reporting of mechanical tests and complies with the NBR, ABNT, ISO, ASTM, and DIN standards [122].
For dynamic fatigue tests, two MTS (MTS Corp., Minneapolis, MN, USA) chewing simulators were used, the MTS Bionix 858 [123, 124] and the MTS Land-mark 370.10 model [125]. MTS test machines feature hydraulic servo innovations, versatile flextest control, and application software that provides high accuracy and reproducibility in high fidelity static and dynamic testing and different types of stress, compression and fatigue tests and comparisons. All comply with ASTM D3039 standards, ASTM D2344, ASTM D790, ASTM D3518, ASTM D5023, ASTM D5024, ASTM D5026, ASTM D5418, ASTM D5992, ASTM D6272, ASTM D7028, ASTM E21, ASTM E290, ASTM E517, ASTM E646, ASTM E8-E8M, ASTM E9, ISO 10113, ISO 10275, ISO 14125, ISO 6892-1, ISO 6892-2, ISO 7438, ISO 14129, ISO 527-4, ISO 527-5, ISO 6721-4, ISO 6721-12, EN 2561, EN 2562, EN 2597, EN 2746, and EN 6031 [126].
The Mastication Simulator MOD (Esetron Smart Robotechnologies, Ankara, Turkey), used in 6 studies [127-131], is a dynamic simulator that has a user-friendly interface and allows remote monitoring during testing that allows adjustment of all test parameters, including temperature. This equipment can be used for mono- or biaxial tests and allows reproducibility of the tests. This simulator is ideal for testing the physical properties of restorative materials or studying the impact of any event on dentition. Esetron test machines comply with ISO 9,100 ISO 12,100 [132].
ADL-Force 5 (Advanced Mechanical Technology Inc., Watertown, MA, USA) was used for static mechanical fatigue testing [124]. Force 5 could replicate the loading and full amplitude of multiaxis motion associated with daily activities. Four servohydraulic actuators control the movement of the mobile base through an electric motor. According to the manufacturer, the test machine complies with the following standards: ISO-14242-1, ASTM-WK451, ASTM-F1612-95, ISO/DIS-7206-4, ISO-14243-1, ISO-14243-3, ASTM- F1715-00, ASTM-F1800-04, ASTM-F1223-05, ASTM-F2423-05, ISO/DIS-12189, ISO/DIS-18192-1, and ASTM-F1717-04 [133].
Chewing simulators manufactured by Lloyd Instruments Ltd. (Fareham, England) have been used, the model LRX [134] and the model LR30K [135], which comply with the ASTM D790, ISO 178, and BS 2782 standards for flexural polymer testing. According to the technical data, this equipment provides high accuracy and reproducibility. For flexural testing, the machines can compensate for the deflection of the equipment [136].
All data presented and discussed here from now on were based on the information reported in the articles. It is important to bear in mind that other testing machines may have the systems or devices we were looking for, but since their presence was not reported in the collected articles, we will consider that these other testing machines were absent.
3. Results
In relation to chewing simulators for the research of the effect of chewing on the food bolus found in 4 studies, 1 of these studies used a commercial chewing simulator from SD Mechatronik manufactured in compliance with standards and with control and monitoring devices [14]. In two studies, the same simulator was used [12, 13] and no monitoring and control devices or systems were reported. In one of the studies, a chewing simulator was used, and although there was no report of devices or monitoring and control systems, the equipment was validated regarding the grinding capacity of the food bolus [32, 34] (Table 1).
All customized simulators were able to produce the experimental conditions of the commercialized types. When we considered the control systems or devices to maintain the same conditions throughout the entire experiment, eight studies reported the precision, frequency, and load control system for the simulator [35-40, 42, 43]. Only one article did not report precision or load control systems [38], and two did not report frequency control [16, 41] (Table 2).
Table 2Information about the accuracy and reproducibility of customized chewing simulators reported in the articles
Reference | Precision control system/mechanism | Frequency control system/mechanism | Load control system/mechanism | Standards reported |
31 | Yes | Yes | Yes | No |
32 | Yes | Yes | Yes | No |
33 | Yes | Yes | Yes | No |
34 | No | Yes | No | No |
35 | Yes | Yes | Yes | No |
36 | Yes | Yes | Yes | No |
37 | Yes | Yes | Yes | No |
38 | Yes | Yes | Yes | Yes |
39 | Yes | Yes | Yes | No |
40,41 | Yes | No | Yes | No |
Among the 82 studies recovered that used chewing simulators commercialized to study the physical properties of materials, 4 used 2 distinct simulators for static and dynamic load [56, 62, 99, 124]. In the present review, commercialized simulators manufactured by Willytec, Mechatronik GmbH, Instron, MTS Corp, Robota Company, eGo Kältesysteme GmbH, Zwick/Roell, Shimadzu, Biopdi, Esetron Smart Robotechnologies, Loyd and ADL (Advanced Mechanical Technology) were found. The most frequently found types were those with Willytec technology (Willytec, SD Mechatronik, eGo Kältresysteme). Regarding the manufacturers, SD Mechatronik simulators were the type most used (Table 3).
4. Discussions
Relative to the customized simulators for the research of the physical properties of biomaterials, 11 studies were found, and only one [43] reported standards for the simulator. The data about the customized chewing simulator developed by the University of Zurich [35, 36] were first published in 1999 [137]. We could not recover complete text, but in the abstract, they reported that it is a computer-controlled simulator, so we inferred that it had a system for monitoring and controlling the experiment.
Table 3Information obtained on the websites of manufacturers about chewing simulators
Reported by the manufacturer | Number of Articles | Control Systems reported | Standards reported |
Willytec | 6 | Yes | Yes** |
Mechatronik GmbH | 33 | Yes | Yes |
Instron | 9 | Yes | Yes |
MTS Corp | 3 | Yes | Yes |
eGo Kältersysteme GmbH | 6 | Yes** | Yes** |
Lloyd Instruments Ltd | 2 | Yes | Yes |
Advanced Mechanical Technology Inc | 1 | Yes | Yes |
Esetron Smart Robotechnologies | 4 | Yes | Yes |
Shimadzu | 1 | Yes | Yes |
Biopdi | 2 | Yes | Yes* |
Robota Company | 1 | Yes | No |
Zwick/Roell | 16 | Yes | Yes |
* No report of standard number ** Since Willytech and Mechatronik technology are the same testing machines changing only the trade mark without technological modification and Ego Kältersysteme uses Willytec technology, although no information about monitoring and controlling system was available on the website, Ego Kältersysteme will be treated as Mechatronik regarding these itens. |
Only two manufacturers (eGo Kältersysteme and Robota Company) did not show any references to their respective sites regarding technical standards for the construction of the simulators. Since eGo Kältesysteme used Wilytec technology, we assumed that the simulators would comply with technical standards. Only the eGo Kältesysteme did not mention a monitoring and controlling system that would guarantee the reproducibility and accuracy of the tests; since they used Willytec technology, we assumed that the simulators would have monitoring and controlling systems.
Based on the data obtained in this review, we believe the testing machines themselves are not responsible for the disparate results found in the literature about the physical properties of biomaterials used for restorative purposes. This finding is in accordance with the literature indicating that, in addition to the test machine, test conditions with, for instance, the number of fatigue cycles [130], load applied [130], thermal cycling [24, 25], environmental conditions [16, 26-28, 130], and wear mechanism [20-23, 130] are factors that influence the test results; therefore, the test conditions are more likely to influence the results currently than the testing machines.
When considering the ISO standards (https://www.iso.org/home.html), ASTM standards (https://www.astm.org/), European standards (https://www.en-standard.eu/), British standards (https://www.bsigroup.com/en-GB/) and Japanese standards (https://webdesk.jsa.or.jp/) used by the test machine manufacturers, it was clear that the testing machines were capable of performing the tests required for dental materials. The standards comprised 23 different physical tests, with 17 different types of materials according to the standards.
Based on the findings of this review, the dentist can be sure of the quality of the material used to restore the occlusal plane and that the material indicated and handled correctly will have minimum influence in stomatognathic functions, always emphasizing that enamel is the best material one can have in the occlusal surface of the teeth.
5. Conclusions
Based on the simulators studied in this review, the following conclusions can be made:
The chewing simulators used for the study of food properties reported systems for controlling and monitoring the experiment.
The majority of the customized chewing simulators appeared to be able to maintain and reproduce the experimental conditions throughout the experimental period.
The commercialized chewing simulators that we found had systems that guaranteed the accuracy and reproducibility of the experiments.
Almost all commercialized chewing simulator manufacturers (except one) showed standards on the website, which guaranteed the accuracy and reproducibility of the experiments.
Based on the data acquired, it would appear that differences in the test results of the physical properties of the materials could be more related to the parameters established for the test than to the accuracy and reproducibility of the chewing simulators or the correct choice of the chewing simulators for the investigation.
The findings suggest that dental material used for occlusal plane restoration do not impact mastication efficiency.
References
-
M. A. Lamb, “Monthly bibliography of dental literature,” The Dental Cosmos, Vol. 37, pp. 466–468, 1895.
-
E. Yilmaz and R. Sadeler, “A literature review on chewing simulation and wear mechanisms of dental biomaterials,” Journal of Bio- and Tribo-Corrosion, Vol. 7, No. 3, pp. 1–10, Sep. 2021, https://doi.org/10.1007/s40735-021-00529-0
-
G. V. Black, “An investigation of the physical characters of the human teeth in relation to their diseases, and to practical dental operations together with the physical characters of filling materials,” The Dental Cosmos, Vol. 37, pp. 469–484, 1895.
-
J. Ahlgren, “Mechanism of mastication,” Acta Odontologica Scandinavica, Vol. 24, pp. 1–109, 1966.
-
J. Ahlgren and B. Öwall, “Muscular activity and chewing force: A polygraphic study of human mandibular movements,” Archives of Oral Biology, Vol. 15, No. 4, pp. 271–IN1, Apr. 1970, https://doi.org/10.1016/0003-9969(70)90053-1
-
S. Koizumi, S. Minamisawa, K. Sasaguri, M. Onozuka, S. Sato, and Y. Ono, “Chewing reduces sympathetic nervous response to stress and prevents poststress arrhythmias in rats,” American Journal of Physiology-Heart and Circulatory Physiology, Vol. 301, No. 4, pp. H1551–H1558, Oct. 2011, https://doi.org/10.1152/ajpheart.01224.2010
-
S. Miyake et al., “Chewing ameliorates stress-induced suppression of spatial memory by increasing glucocorticoid receptor expression in the hippocampus,” Brain Research, Vol. 1446, pp. 34–39, Mar. 2012, https://doi.org/10.1016/j.brainres.2012.01.011
-
C. Ohkubo, M. Morokuma, Y. Yoneyama, R. Matsuda, and J. S. Lee, “Interactions between occlusion and human brain function activities,” Journal of Oral Rehabilitation, Vol. 40, No. 2, pp. 119–129, Feb. 2013, https://doi.org/10.1111/j.1365-2842.2012.02316.x
-
Y. Fukushima-Nakayama et al., “Reduced mastication impairs memory function,” Journal of Dental Research, Vol. 96, No. 9, pp. 1058–1066, Aug. 2017, https://doi.org/10.1177/0022034517708771
-
Y.T. Jou, “Dental deafferentation and brain damage: A review and a hypothesis,” The Kaohsiung Journal of Medical Sciences, Wiley, 2018.
-
J. Saruta, M. To, W. Sakaguchi, Y. Kondo, and K. Tsukinoki, “Brain-derived neurotrophic factor is related to stress and chewing in saliva and salivary glands,” Elsevier BV, Japanese Dental Science Review, Nov. 2020.
-
M. E. C. Menis-Henrique et al., “Cheese-flavored expanded snacks with low lipid content: Oil effects on the in vitro release of butyric acid and on the duration of the dominant sensations of the products,” LWT, Vol. 105, pp. 30–36, May 2019, https://doi.org/10.1016/j.lwt.2019.01.052
-
A. Tarrega, C. Yven, E. Semon, P. Mielle, and C. Salles, “Effect of oral physiology parameters on in-mouth aroma compound release using lipoprotein matrices: an in vitro approach,” Foods, Vol. 8, No. 3, p. 106, Mar. 2019, https://doi.org/10.3390/foods8030106
-
N. Mohammadi, M. R. Ehsani, and H. Bakhoda, “Design and evaluation of the release characteristics of caffeine-loaded microcapsules in a medicated chewing gum formulation,” Food Biophysics, Vol. 13, No. 3, pp. 240–249, Sep. 2018, https://doi.org/10.1007/s11483-018-9530-y
-
S. D. Heintze, A. Eser, D. Monreal, and V. Rousson, “Using a chewing simulator for fatigue testing of metal ceramic crowns,” Journal of the Mechanical Behavior of Biomedical Materials, Vol. 65, pp. 770–780, Jan. 2017, https://doi.org/10.1016/j.jmbbm.2016.09.002
-
E. Yilmaz, R. Sadeler, Z. Duymuş, and A. Özdoğan, “Effect of ambient pH and different chewing cycle of contact wear on dental composite material,” Dentistry and Medical Research, Vol. 6, No. 2, p. 46, 2018, https://doi.org/10.4103/dmr.dmr_26_18
-
S. Heintze, “How to qualify and validate wear simulation devices and methods,” Dental Materials, Vol. 22, No. 8, pp. 712–734, Aug. 2006, https://doi.org/10.1016/j.dental.2006.02.002
-
“.” ZwickRoell. https://www.zwickroell.com
-
J. Head, “The human skull used as a gnathodynamometer to determine the value of trituration in the mastication of food,” The Dental Cosmos, Vol. 18, pp. 1189–1192, 1906.
-
E. Yilmaz and R. Sadeler, “Investigation of two- and three-body wear resistance on flowable bulk-fill and resin-based composites,” Mechanics of Composite Materials, Vol. 54, No. 3, pp. 395–402, Jul. 2018, https://doi.org/10.1007/s11029-018-9750-8
-
E. Yilmaz, “Investigation of two-body wear behavior of zirconia-reinforced lithium silicate glass-ceramic for biomedical applications; in vitro chewing simulation,” Computer Methods in Biomechanics and Biomedical Engineering, Vol. 24, No. 7, pp. 806–815, May 2021, https://doi.org/10.1080/10255842.2020.1852555
-
E. Yilmaz, “Investigation of three‐body wear behavior and hardness of experimental titanium alloys for dental applications in oral environment,” Materialwissenschaft und Werkstofftechnik, Vol. 51, No. 1, pp. 47–53, Jan. 2020, https://doi.org/10.1002/mawe.201900033
-
E. Yilmaz, “Effect of sliding wear mechanism condition on the wear behavior of dental biocomposite materials,” Dentistry and Medical Research, Vol. 8, No. 1, p. 18, 2020, https://doi.org/10.4103/dmr.dmr_2_20
-
R. V. Mesquita and J. Geis-Gerstorfer, “Influence of temperature on the visco-elastic properties of direct and indirect dental composite resins,” Dental Materials, Vol. 24, No. 5, pp. 623–632, May 2008, https://doi.org/10.1016/j.dental.2007.06.025
-
E. Yilmaz and R. Sadeler, “Effect of thermal cycling and microhardness on roughness of composite restorative materials,” Journal of Restorative Dentistry, Vol. 4, No. 3, p. 93, 2016, https://doi.org/10.4103/2321-4619.188233
-
E. Yilmaz, “Effect of contact load upon attrition-corrosion wear behavior of bio-composite materials: In vitro off-axis sliding contact-chewing simulation,” Biomedical Research Journal, Vol. 7, No. 1, p. 17, 2020, https://doi.org/10.4103/bmrj.bmrj_2_20
-
E. Yilmaz, “Effects of thermal change and third-body media particle on wear behaviour of dental restorative composite materials,” Materials Technology, Vol. 34, No. 11, pp. 645–651, Sep. 2019, https://doi.org/10.1080/10667857.2019.1611201
-
E. C. Yilmaz, “Investigation of two-body wear resistance of composite materials for biomaterial application in oral environment: the influence of antagonist material,” Materials Technology, Vol. 35, No. 3, pp. 159–167, Feb. 2020, https://doi.org/10.1080/10667857.2019.1660476
-
L. Sabri, F. Hussein, K. Salloomi, B. Abdulrahman, and A. Al-Zahawi, “Effect of thread depth and implant shape on stress distribution in anterior and posterior regions of mandible bone: A finite element analysis,” Dental Research Journal, Vol. 16, No. 3, p. 200, 2019, https://doi.org/10.4103/1735-3327.255745
-
M. Prados-Privado, S. A. Gehrke, R. Rojo, and J. C. Prados-Frutos, “Complete mechanical characterization of an external hexagonal implant connection: in vitro study, 3D FEM, and probabilistic fatigue,” Medical and Biological Engineering and Computing, Vol. 56, No. 12, pp. 2233–2244, Dec. 2018, https://doi.org/10.1007/s11517-018-1846-8
-
C. G. Skamniotis, M. Elliott, and M. N. Charalambides, “On modelling the constitutive and damage behaviour of highly non-linear bio-composites – Mesh sensitivity of the viscoplastic-damage law computations,” International Journal of Plasticity, Vol. 114, pp. 40–62, Mar. 2019, https://doi.org/10.1016/j.ijplas.2018.10.001
-
M.-A. Peyron et al., “Addressing various challenges related to food bolus and nutrition with the AM2 mastication simulator,” Food Hydrocolloids, Vol. 97, p. 105229, Dec. 2019, https://doi.org/10.1016/j.foodhyd.2019.105229
-
C. Salles et al., “Development of a chewing simulator for food breakdown and the analysis of in vitro flavor compound release in a mouth environment,” Journal of Food Engineering, Vol. 82, No. 2, pp. 189–198, Sep. 2007, https://doi.org/10.1016/j.jfoodeng.2007.02.008
-
A. Woda et al., “Development and validation of a mastication simulator,” Journal of Biomechanics, Vol. 43, No. 9, pp. 1667–1673, Jun. 2010, https://doi.org/10.1016/j.jbiomech.2010.03.002
-
A. Ender, S. Bienz, W. Mörmann, A. Mehl, T. Attin, and B. Stawarczyk, “Marginal adaptation, fracture load and macroscopic failure mode of adhesively luted PMMA-based CAD/CAM inlays,” Dental Materials, Vol. 32, No. 2, pp. e22–e29, Feb. 2016, https://doi.org/10.1016/j.dental.2015.11.009
-
L. Zhi, T. Bortolotto, and I. Krejci, “Comparative in vitro wear resistance of CAD/CAM composite resin and ceramic materials,” The Journal of Prosthetic Dentistry, Vol. 115, No. 2, pp. 199–202, Feb. 2016, https://doi.org/10.1016/j.prosdent.2015.07.011
-
W. Singhatanadgit, P. Junkaew, and P. Singhatanadgid, “Effect of bidirectional loading on contact and force characteristics under a newly developed masticatory simulator with a dual-direction loading system,” Dental Materials Journal, Vol. 35, No. 6, pp. 952–961, 2016, https://doi.org/10.4012/dmj.2016-198
-
Yamini Gundugollu, R. Yalavarthy, M. Krishna, S. Kalluri, Shameen Kumar Pydi, and Satyendra Kumar Tedlapu, “Comparison of the effect of monolithic and layered zirconia on natural teeth wear: An in vitro study,” The Journal of the Indian Prosthodontic Society, Vol. 18, pp. 336–342, 2018.
-
J. Guo et al., “Effect of contact stress on the cycle-dependent wear behavior of ceramic restoration,” Journal of the Mechanical Behavior of Biomedical Materials, Vol. 68, pp. 16–25, Apr. 2017, https://doi.org/10.1016/j.jmbbm.2017.01.027
-
H. Zipprich, S. Miatke, R. Hmaidouch, and H.-C. Lauer, “A new experimental design for bacterial microleakage investigation at the implant-abutment interface: an in vitro study,” The International Journal of Oral and Maxillofacial Implants, Vol. 31, No. 1, pp. 37–44, Jan. 2016, https://doi.org/10.11607/jomi.3713
-
H. Zipprich, “Micro-movements and micro-pump effect of implant-abutment-connection 2010. Test-Report for Konus K3Pro Dental Implants,” http://www.argon-dental.de/images/studien/micro-movements-and-micro-pump-effect-of-implant-abutment-connection-report.pdf, 2010.
-
C. Dayan, B. Kiseri, B. Gencel, H. Kurt, and N. Tuncer, “Wear resistance and microhardness of various interim fixed prosthesis materials,” Journal of Oral Science, Vol. 61, No. 3, pp. 447–453, 2019, https://doi.org/10.2334/josnusd.18-0323
-
A. M. Tahir, M. Jilich, D. C. Trinh, G. Cannata, F. Barberis, and M. Zoppi, “Architecture and design of a robotic mastication simulator for interactive load testing of dental implants and the mandible,” The Journal of Prosthetic Dentistry, Vol. 122, No. 4, pp. 389.e1–389.e8, Oct. 2019, https://doi.org/10.1016/j.prosdent.2019.06.023
-
K. K. C. Ip, P. You, C. C. Moore, and L. M. Ferreira, “Bite force simulator: a novel technique to simulate craniofacial strain in vitro,” Journal of Craniofacial Surgery, Vol. 31, No. 3, pp. 838–842, May 2020, https://doi.org/10.1097/scs.0000000000006091
-
E. Yilmaz, R. Sadeler, Z. Duymuş, and M. Öcal, “Effects of two-body wear on microfill, nanofill, and nanohybrid restorative composites,” Biomedical and Biotechnology Research Journal (BBRJ), Vol. 1, No. 1, p. 25, 2017, https://doi.org/10.4103/bbrj.bbrj_36_17
-
N. Passia, M. Ghazal, and M. Kern, “Long-term retention behaviour of resin matrix attachment systems for overdentures,” Journal of the Mechanical Behavior of Biomedical Materials, Vol. 57, pp. 88–94, Apr. 2016, https://doi.org/10.1016/j.jmbbm.2015.11.038
-
C. Yazigi, M. Kern, and M. S. Chaar, “Influence of various bonding techniques on the fracture strength of thin CAD/CAM-fabricated occlusal glass-ceramic veneers,” Journal of the Mechanical Behavior of Biomedical Materials, Vol. 75, pp. 504–511, Nov. 2017, https://doi.org/10.1016/j.jmbbm.2017.08.016
-
M. V. Swain, A. Coldea, A. Bilkhair, and P. C. Guess, “Interpenetrating network ceramic-resin composite dental restorative materials,” Dental Materials, Vol. 32, No. 1, pp. 34–42, Jan. 2016, https://doi.org/10.1016/j.dental.2015.09.009
-
M. Al-Akhali, M. S. Chaar, A. Elsayed, A. Samran, and M. Kern, “Fracture resistance of ceramic and polymer-based occlusal veneer restorations,” Journal of the Mechanical Behavior of Biomedical Materials, Vol. 74, pp. 245–250, Oct. 2017, https://doi.org/10.1016/j.jmbbm.2017.06.013
-
A. Alsahhaf, B. C. Spies, K. Vach, and R.-J. Kohal, “Fracture resistance of zirconia-based implant abutments after artificial long-term aging,” Journal of the Mechanical Behavior of Biomedical Materials, Vol. 66, pp. 224–232, Feb. 2017, https://doi.org/10.1016/j.jmbbm.2016.11.018
-
C. Yazigi, H. Schneider, M. S. Chaar, C. Rüger, R. Haak, and M. Kern, “Effects of artificial aging and progression of cracks on thin occlusal veneers using SD-OCT,” Journal of the Mechanical Behavior of Biomedical Materials, Vol. 88, pp. 231–237, Dec. 2018, https://doi.org/10.1016/j.jmbbm.2018.08.017
-
V. Preis, A. Kammermeier, G. Handel, and M. Rosentritt, “In vitro performance of two-piece zirconia implant systems for anterior application,” Dental Materials, Vol. 32, No. 6, pp. 765–774, Jun. 2016, https://doi.org/10.1016/j.dental.2016.03.028
-
M. Naumann, M. Von Stein-Lausnitz, M. Rosentritt, C. Walter, H. Meyer-Lückel, and G. Sterzenbach, “Impact of simulated reduced alveolar bone support, increased tooth mobility, and distal post-supported, root-treated abutment tooth on load capability of all-ceramic zirconia-supported cantilever FDP,” Clinical Oral Investigations, Vol. 22, No. 8, pp. 2799–2807, Nov. 2018, https://doi.org/10.1007/s00784-018-2366-5
-
V. Preis, S. Hahnel, M. Behr, and M. Rosentritt, “In vitro performance and fracture resistance of novel CAD/CAM ceramic molar crowns loaded on implants and human teeth,” The Journal of Advanced Prosthodontics, Vol. 10, No. 4, pp. 300–307, 2018, https://doi.org/10.4047/jap.2018.10.4.300
-
A. Kammermeier, M. Rosentritt, M. Behr, S. Schneider-Feyrer, and V. Preis, “In vitro performance of one – and two-piece zirconia implant systems for anterior application,” Journal of Dentistry, Vol. 53, pp. 94–101, Oct. 2016, https://doi.org/10.1016/j.jdent.2016.08.004
-
M. Von Stein-Lausnitz et al., “Direct restoration of endodontically treated maxillary central incisors: post or no post at all?,” Clinical Oral Investigations, Vol. 23, No. 1, pp. 381–389, Jan. 2019, https://doi.org/10.1007/s00784-018-2446-6
-
M. Rosentritt, F. Schumann, S. Krifka, and V. Preis, “Influence of zirconia and lithium disilicate tooth – or implant-supported crowns on wear of antagonistic and adjacent teeth,” The Journal of Advanced Prosthodontics, Vol. 12, No. 1, pp. 1–8, 2020, https://doi.org/10.4047/jap.2020.12.1.1
-
eGo-Kältesysteme, https://www.ego-kaeltesysteme.de
-
SD Mechatronik, https://sd-mechatronik.de
-
J.-W. Choi et al., “Wear of primary teeth caused by opposed all-ceramic or stainless steel crowns,” The Journal of Advanced Prosthodontics, Vol. 8, No. 1, pp. 43–52, 2016, https://doi.org/10.4047/jap.2016.8.1.43
-
T. Koutouzis, H. Gadalla, and T. Lundgren, “Bacterial colonization of the implant-abutment interface (iai) of dental implants with a sloped marginal design: An in-vitro Study,” Clinical Implant Dentistry and Related Research, Vol. 18, No. 1, pp. 161–167, Feb. 2016, https://doi.org/10.1111/cid.12287
-
K. Ladetzki, R. Mateos-Palacios, A. Pascual-Moscardo, and E. Selva-Otaolaurruchi, “Effect of retention design of artificial teeth and implant-supported titanium CAD-CAM structures on fracture resistance,” Journal of Clinical and Experimental Dentistry, Vol. 8, 2016, https://doi.org/10.4317/jced.52228
-
K.-J. Yoon, Y.-B. Park, H. Choi, Y. Cho, J.-H. Lee, and K.-W. Lee, “Evaluation of stability of interface between CCM (Co-Cr-Mo) UCLA abutment and external hex implant,” The Journal of Advanced Prosthodontics, Vol. 8, No. 6, pp. 465–471, 2016, https://doi.org/10.4047/jap.2016.8.6.465
-
H. Askar, F. Brouwer, M. Lehmensiek, S. Paris, and F. Schwendicke, “The association between loading of restorations and secondary caries lesions is moderated by the restoration material elasticity,” Journal of Dentistry, Vol. 58, pp. 74–79, Mar. 2017, https://doi.org/10.1016/j.jdent.2017.01.002
-
A. Elsayed, G. Meyer, S. Wille, and M. Kern, “Influence of the yttrium content on the fracture strength of monolithic zirconia crowns after artificial aging,” Quintessence International, Vol. 50, No. 5, pp. 344–348, Apr. 2019, https://doi.org/10.3290/j.qi.a42097
-
S.-M. Im, Y.-H. Huh, L.-R. Cho, and C.-J. Park, “Comparison of the fracture resistances of glass fiber mesh – and metal mesh-reinforced maxillary complete denture under dynamic fatigue loading,” The Journal of Advanced Prosthodontics, Vol. 9, No. 1, pp. 22–30, 2017, https://doi.org/10.4047/jap.2017.9.1.22
-
Fariborz Vafaee, Farnaz Firouz, Masoumeh Khoshhal, Amirarsalan Hooshyarfard, Armaghan Shahbazi, and Ghodratollah Roshanaei, “Fatigue fracture strength of implant-supported full contour zirconia and metal ceramic fixed partial dentures,” Journal of Dentistry (Tehran, Iran), Vol. 14, No. 3, p. 165, May 2017.
-
C. D. ’Arcangelo, L. Vanini, G. Rondoni, M. Vadini, and F. de Angelis, “Wear evaluation of prosthetic materials opposing themselves,” Operative Dentistry, Vol. 43, No. 1, pp. 38–50, Jan. 2018, https://doi.org/10.2341/16-212-l
-
S. H. Elshiyab, N. Nawafleh, L. Walsh, and R. George, “Fracture resistance and survival of implant-supported, zirconia-based hybrid-abutment crowns: Influence of aging and crown structure,” Journal of Investigative and Clinical Dentistry, Vol. 9, No. 4, p. e12355, Nov. 2018, https://doi.org/10.1111/jicd.12355
-
S. Garoushi, P. K. Vallittu, and L. Lassila, “Characterization of fluoride releasing restorative dental materials,” Dental Materials Journal, Vol. 37, No. 2, pp. 293–300, 2018, https://doi.org/10.4012/dmj.2017-161
-
Irmak, B. C. Yaman, D. Y. Lee, E. O. Orhan, F. K. Mante, and F. Ozer, “Flexural strength of fiber reinforced posts after mechanical aging by simulated chewing forces,” Journal of the Mechanical Behavior of Biomedical Materials, Vol. 77, pp. 135–139, Jan. 2018, https://doi.org/10.1016/j.jmbbm.2017.09.001
-
T. Kewekordes, S. Wille, and M. Kern, “Wear of polyetherketoneketones – Influence of titanium dioxide content and antagonistic material,” Dental Materials, Vol. 34, No. 3, pp. 560–567, Mar. 2018, https://doi.org/10.1016/j.dental.2017.12.009
-
M. Lehmensiek, H. Askar, F. Brouwer, U. Blunck, S. Paris, and F. Schwendicke, “Restoration integrity, but not material or cementation strategy determined secondary caries lesions next to indirect restorations in vitro,” Dental Materials, Vol. 34, No. 12, pp. e317–e323, Dec. 2018, https://doi.org/10.1016/j.dental.2018.09.004
-
N. Nawafleh, M. M. Hatamleh, A. Öchsner, and F. Mack, “The impact of core/veneer thickness ratio and cyclic loading on fracture resistance of lithium disilicate crown,” Journal of Prosthodontics, Vol. 27, No. 1, pp. 75–82, Jan. 2018, https://doi.org/10.1111/jopr.12473
-
J. Roulet, N. Abdulhameed, and Chiayi Shen, “In vitro wear of three bulk fill composites and enamel,” Stomatology Education Journal, pp. 248–253, 2017.
-
F. Santos, A. Branco, M. Polido, A. P. Serro, and C. G. Figueiredo-Pina, “Comparative study of the wear of the pair human teeth/Vita Enamic® vs commonly used dental ceramics through chewing simulation,” Journal of the Mechanical Behavior of Biomedical Materials, Vol. 88, pp. 251–260, Dec. 2018, https://doi.org/10.1016/j.jmbbm.2018.08.029
-
I. Sarıkaya and Y. Hayran, “Effects of dynamic aging on the wear and fracture strength of monolithic zirconia restorations,” BMC Oral Health, Vol. 18, No. 1, pp. 1–7, Dec. 2018, https://doi.org/10.1186/s12903-018-0618-z
-
T. Q. Qasim, B. M. El-Masoud, and A. M. A. Laban, “The effect of resistance grooves on the fracture toughness of zirconia-based crowns from mono and cyclic loading,” European Journal of Dentistry, Vol. 12, No. 4, pp. 491–495, Oct. 2018, https://doi.org/10.4103/ejd.ejd_207_18
-
K. Zierden, J. Acar, P. Rehmann, and B. Wöstmann, “Wear and fracture strength of new ceramic resins for chairside milling,” The International Journal of Prosthodontics, Vol. 31, No. 1, pp. 74–76, Jan. 2018, https://doi.org/10.11607/ijp.5492
-
R. Agustín-Panadero, B. Serra-Pastor, A. Roig-Vanaclocha, A. Fons-Font, and M. F. Solá-Ruiz, “Fracture resistance and the mode of failure produced in metal-free crowns cemented onto zirconia abutments in dental implants,” PLOS ONE, Vol. 14, No. 8, p. e0220551, Aug. 2019, https://doi.org/10.1371/journal.pone.0220551
-
S. Atsü, M. Aksan, and A. Bulut, “Fracture resistance of titanium, zirconia, and ceramic-reinforced polyetheretherketone implant abutments supporting CAD/CAM monolithic lithium disilicate ceramic crowns after aging,” The International Journal of Oral and Maxillofacial Implants, Vol. 34, No. 3, pp. 622–630, May 2019, https://doi.org/10.11607/jomi.7036
-
L. Lassila, E. Säilynoja, R. Prinssi, P. Vallittu, and S. Garoushi, “Characterization of a new fiber-reinforced flowable composite,” Odontology, Vol. 107, No. 3, pp. 342–352, Jul. 2019, https://doi.org/10.1007/s10266-018-0405-y
-
P. Baumgart, H. Kirsten, R. Haak, and C. Olms, “Biomechanical properties of polymer-infiltrated ceramic crowns on one-piece zirconia implants after long-term chewing simulation,” International Journal of Implant Dentistry, Vol. 4, No. 1, pp. 1–7, Dec. 2018, https://doi.org/10.1186/s40729-018-0127-5
-
S. Dayan and E. Mumcu, “Effect of different storage media on the microhardness and wear resistance of resin‐matrix ceramics,” International Journal of Applied Ceramic Technology, Vol. 16, No. 6, pp. 2467–2473, Nov. 2019, https://doi.org/10.1111/ijac.13307
-
A. Elkabbany, M. Kern, A. H. Elkhadem, S. Wille, A. A. Amer, and M. S. Chaar, “Retention of metallic and non-metallic double-crown-retained mandibular overdentures on implants: An in-vitro study,” Journal of Prosthodontic Research, Vol. 64, No. 4, pp. 384–390, Oct. 2020, https://doi.org/10.1016/j.jpor.2019.11.001
-
A.-M. Lutz, R. Hampe, M. Roos, N. Lümkemann, M. Eichberger, and B. Stawarczyk, “Fracture resistance and 2-body wear of 3-dimensional-printed occlusal devices,” The Journal of Prosthetic Dentistry, Vol. 121, No. 1, pp. 166–172, Jan. 2019, https://doi.org/10.1016/j.prosdent.2018.04.007
-
C. Riedel, M. Wendler, R. Belli, A. Petschelt, and U. Lohbauer, “In vitro lifetime of zirconium dioxide-based crowns veneered using Rapid Layer Technology,” European Journal of Oral Sciences, Vol. 127, No. 2, pp. 179–186, Apr. 2019, https://doi.org/10.1111/eos.12604
-
R. Sorrentino et al., “Effects of finish line design and fatigue cyclic loading on phase transformation of zirconia dental ceramics: a qualitative micro-raman spectroscopic analysis,” Materials, Vol. 12, No. 6, p. 863, Mar. 2019, https://doi.org/10.3390/ma12060863
-
P. Hsu, Ramos, and A. Sadr, “Microcomputed tomography evaluation of cement shrinkage under zirconia versus lithium disilicate veneers,” The Journal of Prosthetic Dentistry, Vol. 125, No. 2, pp. 307–315, Feb. 2021, https://doi.org/10.1016/j.prosdent.2020.01.021
-
S. Zidan, N. Silikas, J. Haider, A. Alhotan, J. Jahantigh, and J. Yates, “Evaluation of equivalent flexural strength for complete removable dentures made of zirconia-impregnated PMMA nanocomposites,” Materials, Vol. 13, No. 11, p. 2580, Jun. 2020, https://doi.org/10.3390/ma13112580
-
B. Stawarczyk, K. Frevert, A. Ender, M. Roos, B. Sener, and T. Wimmer, “Comparison of four monolithic zirconia materials with conventional ones: Contrast ratio, grain size, four-point flexural strength and two-body wear,” Journal of the Mechanical Behavior of Biomedical Materials, Vol. 59, pp. 128–138, Jun. 2016, https://doi.org/10.1016/j.jmbbm.2015.11.040
-
S.-F. Huang, W.-R. Chen, and C.-L. Lin, “Biomechanical interactions of endodontically treated tooth implant-supported prosthesis under fatigue test with acoustic emission monitoring,” BioMedical Engineering OnLine, Vol. 15, No. 1, pp. 22–10, Dec. 2016, https://doi.org/10.1186/s12938-016-0140-y
-
D. Jallal Hadi Mahmood, E. Linderoth, A. Wennerberg, and P. Vult Von Steyern, “Influence of core design, production technique, and material selection on fracture behavior of yttria-stabilized tetragonal zirconia polycrystal fixed dental prostheses produced using different multilayer techniques: split-file, over-pressing, and manually built-up veneers,” Clinical, Cosmetic and Investigational Dentistry, Vol. 8, pp. 15–27, Feb. 2016, https://doi.org/10.2147/ccide.s94343
-
P. A. Pinto, G. Colas, T. Filleter, and G. M. de Souza, “Surface and mechanical characterization of dental yttria-stabilized tetragonal zirconia polycrystals (3Y-TZP) after different aging processes,” Microscopy and Microanalysis, Vol. 22, No. 6, pp. 1179–1188, Dec. 2016, https://doi.org/10.1017/s1431927616011843
-
J.-W. Choi, S.-Y. Kim, J.-H. Bae, E.-B. Bae, and J.-B. Huh, “In vitro study of the fracture resistance of monolithic lithium disilicate, monolithic zirconia, and lithium disilicate pressed on zirconia for three-unit fixed dental prostheses,” The Journal of Advanced Prosthodontics, Vol. 9, No. 4, pp. 244–251, 2017, https://doi.org/10.4047/jap.2017.9.4.244
-
D. Pedrollo Lise, A. van Ende, J. de Munck, T. Y. Umeda Suzuki, L. C. Cardoso Vieira, and B. van Meerbeek, “Biomechanical behavior of endodontically treated premolars using different preparation designs and CAD/CAM materials,” Journal of Dentistry, Vol. 59, pp. 54–61, Apr. 2017, https://doi.org/10.1016/j.jdent.2017.02.007
-
M. R. Alammari, M. H. Abdelnabi, and A. A. Swelem, “Effect of total occlusal convergence on fit and fracture resistance of zirconia-reinforced lithium silicate crowns,” Clinical, Cosmetic and Investigational Dentistry, Vol. Volume 11, pp. 1–8, Dec. 2018, https://doi.org/10.2147/ccide.s193326
-
C. Clausson, C. C. Schroeder, P. V. Goloni, F. A. R. Farias, L. Passos, and R. V. Zanetti, “Fracture resistance of CAD/CAM lithium disilicate of endodontically treated mandibular damaged molars based on different preparation designs,” International Journal of Biomaterials, Vol. 2019, pp. 1–7, May 2019, https://doi.org/10.1155/2019/2475297
-
O. Sagsoz and N. Polat Sagsoz, “Chemical degradation of dental CAD/CAM materials,” Bio-Medical Materials and Engineering, Vol. 30, No. 4, pp. 419–426, Sep. 2019, https://doi.org/10.3233/bme-191063
-
“.” Instron. https://www.instron.com.br/pt-br/?region=brasil
-
Nazari V., Ghodsi S., Alikhasi M., Sahebi M., and Shamshiri Ar, “Fracture strength of three-unit implant supported fixed partial dentures with excessive crown height fabricated from different materials,” Journal of dentistry (Tehran, Iran), Vol. 13, No. 6, pp. 400–406, Nov. 2016.
-
B. Stawarczyk, A. Liebermann, M. Eichberger, and J.-F. Güth, “Evaluation of mechanical and optical behavior of current esthetic dental restorative CAD/CAM composites,” Journal of the Mechanical Behavior of Biomedical Materials, Vol. 55, pp. 1–11, Mar. 2016, https://doi.org/10.1016/j.jmbbm.2015.10.004
-
S. Habibzadeh, H. R. Rajati, H. Hajmiragha, S. Esmailzadeh, and M. Kharazifard, “Fracture resistances of zirconia, cast Ni-Cr, and fiber-glass composite posts under all-ceramic crowns in endodontically treated premolars,” The Journal of Advanced Prosthodontics, Vol. 9, No. 3, pp. 170–175, 2017, https://doi.org/10.4047/jap.2017.9.3.170
-
K. Sieper, S. Wille, and M. Kern, “Fracture strength of lithium disilicate crowns compared to polymer-infiltrated ceramic-network and zirconia reinforced lithium silicate crowns,” Journal of the Mechanical Behavior of Biomedical Materials, Vol. 74, pp. 342–348, Oct. 2017, https://doi.org/10.1016/j.jmbbm.2017.06.025
-
A. R. Alkharrat, M. Schmitter, S. Rues, and P. Rammelsberg, “Fracture behavior of all-ceramic, implant-supported, and tooth-implant-supported fixed dental prostheses,” Clinical Oral Investigations, Vol. 22, No. 4, pp. 1663–1673, May 2018, https://doi.org/10.1007/s00784-017-2233-9
-
A. Elsayed, S. Wille, M. Al-Akhali, and M. Kern, “Effect of fatigue loading on the fracture strength and failure mode of lithium disilicate and zirconia implant abutments,” Clinical Oral Implants Research, Vol. 29, No. 1, pp. 20–27, Jan. 2018, https://doi.org/10.1111/clr.13034
-
M. Obermeier, O. Ristow, K. Erdelt, and F. Beuer, “Mechanical performance of cement – and screw-retained all-ceramic single crowns on dental implants,” Clinical Oral Investigations, Vol. 22, No. 2, pp. 981–991, Mar. 2018, https://doi.org/10.1007/s00784-017-2178-z
-
S. Bishti, C. Jäkel, M. Kern, and S. Wolfart, “Influence of different preparation forms on the loading-bearing capacity of zirconia cantilever FDPs. A laboratory study,” Journal of Prosthodontic Research, Vol. 63, No. 3, pp. 347–353, Jul. 2019, https://doi.org/10.1016/j.jpor.2018.10.010
-
S. Jagodin, M. Sasse, S. Freitag-Wolf, and M. Kern, “Influence of attachment design and material on the retention of resin-bonded attachments,” Clinical Oral Investigations, Vol. 23, No. 3, pp. 1217–1223, Mar. 2019, https://doi.org/10.1007/s00784-018-2544-5
-
M. Kelch, J. Schulz, D. Edelhoff, B. Sener, and B. Stawarczyk, “Impact of different pretreatments and aging procedures on the flexural strength and phase structure of zirconia ceramics,” Dental Materials, Vol. 35, No. 10, pp. 1439–1449, Oct. 2019, https://doi.org/10.1016/j.dental.2019.07.020
-
I. Nouh, M. Kern, A. E. Sabet, A. K. Aboelfadl, A. M. Hamdy, and M. S. Chaar, “Mechanical behavior of posterior all-ceramic hybrid-abutment-crowns versus hybrid-abutments with separate crowns-A laboratory study,” Clinical Oral Implants Research, Vol. 30, No. 1, pp. 90–98, Jan. 2019, https://doi.org/10.1111/clr.13395
-
J. Angermair, D. Nolte, R. Linsenmann, and K.H. Kunzelmann, “The influence of storage temperature on fracture behavior of cryopreserved teeth-An in vitro study,” Clinical and Experimental Dental Research, Vol. 6, No. 3, pp. 373–380, Jun. 2020, https://doi.org/10.1002/cre2.283
-
K.-C. Brunner and M. Özcan, “Load bearing capacity and Weibull characteristics of inlay-retained resin-bonded fixed dental prosthesis made of all-ceramic, fiber-reinforced composite and metal-ceramic after cyclic loading,” Journal of the Mechanical Behavior of Biomedical Materials, Vol. 109, p. 103855, Sep. 2020, https://doi.org/10.1016/j.jmbbm.2020.103855
-
A. Loannidis, D. Bomze, C. H. F. Hämmerle, J. Hüsler, O. Birrer, and S. Mühlemann, “Load-bearing capacity of CAD/CAM 3D-printed zirconia, CAD/CAM milled zirconia, and heat-pressed lithium disilicate ultra-thin occlusal veneers on molars,” Dental Materials, Vol. 36, No. 4, pp. e109–e116, Apr. 2020, https://doi.org/10.1016/j.dental.2020.01.016
-
A. Winter, A. Schurig, E. Rasche, F. Rösner, L. Kanus, and M. Schmitter, “The flexural strength of CAD/CAM polymer crowns and the effect of artificial ageing on the fracture resistance of CAD/CAM polymer and ceramic single crowns,” Journal of Materials Science: Materials in Medicine, Vol. 31, No. 1, pp. 1–8, Jan. 2020, https://doi.org/10.1007/s10856-019-6347-2
-
S. M. Fathy and M. V. Swain, “In-vitro wear of natural tooth surface opposed with zirconia reinforced lithium silicate glass ceramic after accelerated ageing,” Dental Materials, Vol. 34, No. 3, pp. 551–559, Mar. 2018, https://doi.org/10.1016/j.dental.2017.12.010
-
Robota industries, https://www.robota-eg.com/chewing-simulator.
-
M. Mieda et al., “The effective design of zirconia coping on titanium base in dental implant superstructure,” Dental Materials Journal, Vol. 37, No. 2, pp. 237–243, 2018, https://doi.org/10.4012/dmj.2017-022
-
Shimadzu, https://www.shimadzu.com/
-
J. A. Sedrez-Porto, E. A. Münchow, L. L. Valente, M. S. Cenci, and T. Pereira-Cenci, “New material perspective for endocrown restorations: effects on mechanical performance and fracture behavior,” Brazilian Oral Research, Vol. 33, p. e012, 2019, https://doi.org/10.1590/1807-3107bor-2019.vol33.0012
-
P. F. D. Silva et al., “Effect of selective carious tissue removal on biomechanical behavior of class II bulk-fill dental composite restorations,” Dental Materials, Vol. 34, No. 9, pp. 1289–1298, Sep. 2018, https://doi.org/10.1016/j.dental.2018.05.014
-
Biopdi, https://biopdi.com.br/
-
T. R. Katona and G. J. Eckert, “The mechanics of dental occlusion and disclusion,” Clinical Biomechanics, Vol. 50, pp. 84–91, Dec. 2017, https://doi.org/10.1016/j.clinbiomech.2017.10.009
-
W. H. Kim et al., “Mechanical assessment of fatigue characteristics between single- and multi-directional cyclic loading modes on a dental implant system,” Materials, Vol. 13, No. 7, p. 1545, Mar. 2020, https://doi.org/10.3390/ma13071545
-
Z. Zhang et al., “Effects of crystal refining on wear behaviors and mechanical properties of lithium disilicate glass-ceramics,” Journal of the Mechanical Behavior of Biomedical Materials, Vol. 81, pp. 52–60, May 2018, https://doi.org/10.1016/j.jmbbm.2018.02.023
-
MTS, https://www.mts.com
-
M. Bankoğlu Güngör and S. Karakoca Nemli, “Fracture resistance of CAD-CAM monolithic ceramic and veneered zirconia molar crowns after aging in a mastication simulator,” The Journal of Prosthetic Dentistry, Vol. 119, No. 3, pp. 473–480, Mar. 2018, https://doi.org/10.1016/j.prosdent.2017.05.003
-
M. Bankoğlu Güngör, S. Karakoca Nemli, H. Yılmaz, and C. Aydın, “Fracture resistance of different implant supported ceramic abutment/crown systems,” European Oral Research, Vol. 53, No. 2, pp. 80–87, May 2019, https://doi.org/10.26650/eor.20199657
-
V. Brzović Rajić, A. Ivanišević Malčić, Z. Bilge Kütük, S. Gurgan, S. Jukić Krmek, and I. Miletić, “Compressive strength of new glass ionomer cement technology based restorative materials after thermocycling and cyclic loading,” Acta Stomatologica Croatica, Vol. 53, No. 4, pp. 318–325, Dec. 2019, https://doi.org/10.15644/asc53/4/2
-
N. Sen and Y. O. Us, “Fatigue survival and failure resistance of titanium versus zirconia implant abutments with various connection designs,” The Journal of Prosthetic Dentistry, Vol. 122, No. 3, pp. 315.e1–315.e7, Sep. 2019, https://doi.org/10.1016/j.prosdent.2019.05.036
-
M. Benli et al., “Surface roughness and wear behavior of occlusal splint materials made of contemporary and high-performance polymers,” Odontology, Vol. 108, No. 2, pp. 240–250, Apr. 2020, https://doi.org/10.1007/s10266-019-00463-1
-
Esetron, http://esetron.com/portfolio/occusal-loading-chewing-simulator
-
AMTI, https://amti.biz/select%20product%20pdfs/simulator%20machines/
-
J. He, S. Garoushi, E. Säilynoja, P. K. Vallittu, and L. Lassila, “The effect of adding a new monomer “Phene” on the polymerization shrinkage reduction of a dental resin composite,” Dental Materials, Vol. 35, No. 4, pp. 627–635, Apr. 2019, https://doi.org/10.1016/j.dental.2019.02.006
-
G. D. ’Addazio et al., “Fracture resistance of zirconia-reinforced lithium silicate ceramic crowns cemented with conventional or adhesive systems: an in vitro study,” Materials, Vol. 13, No. 9, p. 2012, Apr. 2020, https://doi.org/10.3390/ma13092012
-
AMETEK Sensors, https://www.ametektest.com/-/media/ametektest/download_links/lloyd-instruments-materials-testing-catalogue-2020.pdf?la=en
-
Krejci I., Reich T., Lutz F., and Albertoni M., “An in vitro test procedure for evaluating dental restoration systems. 1. A computer-controlled mastication simulator,” Schweiz Monatsschr Zahnmed, Vol. 100, No. 8, pp. 953–960, 1990.
About this article
The authors thank the “National Council for Scientific and Technological Development-CNPq”. Process: 313145/2020-2 for supporting this study.
The datasets generated during and/or analyzed during the current study are available from the corresponding author on reasonable request.
Orlando Santiago Júnior: conceptualization, investigation, methodology, writing original draft, validation. Marcus Vinicius Ferreira: investigation, writing-review and editing, validation. Rudolf Huebner: conceptualization, funding acquisition, supervision, writing-review and editing, validation.
The authors declare that they have no conflict of interest.