Abstract
Golf is an extremely popular activity that over 60 million of people enjoy playing it. Overuse injuries associated with the back, neck, wrist, shoulder, elbow, knee, etc., are common for both professional and amateur player due to golf swing. A lot of researchers investigate the mechanical behavior of golf club or biological response of human body individually. But there is little study on the dynamic interaction between the hand-arm and golf club mashie (HAGCM) when striking ball. Solidworks package is used to draw the 3D geometric model of a left hand-arm contacted at the grip of the golf club. Then the 3D biomechanical model is imported into ANSYS software for transient (impact) analysis. Due to complicated geometry of the sharp edge at the club head and the hand-arm, the tetrahedral element is applied for all components of HAGCM to save computer resource during ANSYS process. Finite element convergence test is obtained before any further analysis. In order to simulate the ball-striking, the hitting surface of the club head is subjected to the impact force of 22 N within 0.01 seconds. All the deformations, principle and shear stresses of the time response found in the vibrating golf head, shaft, grip and hand are compared with each other. Normal and eccentric impact analyses for HAGCM are examined as well. The angular velocity found in eccentric impact is much higher than that in normal impact, and it will cause the muscle to exert more power to hold the golf club.
1. Introduction
Almost in every epoch of history, people played the game with a club and a ball. Golf is mostly played by a group of two to four persons to increase usage of the course. Different golf clubs are used by the player to hit the small ball into the hole. Most of the major tournaments have 72 holes, 4 rounds of 18. The player with the lowest score is declared the winner [1]. A professional golf-player is reported serious sports injuries averagely twice a year [2]. The most common golf injury is the lower back area, followed by the wrists, shoulders, elbows, knees, etc. Those injuries are usually happened in the left side of the body of a right-handed player, and the major factors are swing biomechanical properties and overuse of the body. A complete swing needs to use many joints, links, muscles, etc., involving not only large range of motion but also instant high speed. The classic swing plane, emphasizing the rotation of hip, shoulder and club, is around 60 degree of angle in between with the horizontal plane. In order to obtain a higher club head speed and longer driving distance, modern swing is to relatively limit hip movement, and to change the motions on the back, shoulders and hands to generate effective torque. Hence, the cooperation of hand-arm and golf club is crucial to strike a far, smooth and straight ball.
There are a lot of papers studied on the structural behavior of golf head, shaft and ball. Jaknowan et al. [3] developed a calibration system for golf shaft fitting to the club or blade. This calibration tool was used to determine the best angle for fitting the shaft to the golf club which reflected the minimal swaying while hitting to the ball. Hence, the golfers could control the desired range and direction of the ball. Lacy et al. [4] carefully selected player tests to isolate the coupled influence of club mass and shaft length, typical of those found in currently available drivers, on initial ball velocity, spin rates, launch angle, and dispersion of impacts across the clubface. They found that longer, lighter clubs generally led to higher ball speeds for most test golfers, those combinations of club mass and club length that minimized spin rates resulted in the greatest estimated total shot distance. Hayase et al. [5] investigated the magnitude of the principal strain of the head face through computer simulation and experiments. The bending of the face in the vertical direction was considered to be a major influential factor of the maximum principal strain. Silva et al. [6] analyzed the temporal activity during the golf swing given the preparation phase (backswing) and execution phase (downswing) by comparing the use of two different baselines, activity threshold methods and visual inspection.
Some researchers studied biomechanical response on hand-arm, fingers and palm. Adewusi et al. [7] found that the percentage of power absorbed in different hand-arm substructures was dependent on the operating speed of the power tools, the higher the operating speed the higher the power absorbed in the hand-arm system and vice versa. Dong et al. [8] developed a five degrees-of-freedom (DOF) model to estimate the biodynamic responses distributed at the fingers and the palm of the hand based on the total driving-point mechanical impedance of the entire hand-arm system. The model can also be used to assess the risk of the location-specific disorders induced from the hand-transmitted vibration exposure which will cause hand-arm vibration syndrome (HAVS). One of the major symptoms of HAVS is vibration white finger (VWF) caused by exaggerated vasoconstriction of the arteries and skin arterioles. Pattnaik et al. [9] modeled a small artery as a fluid filled elastic tube whose diameter changes along the axial direction. The modeled artery system shows a spatial resonance as in the basilar membrane, which responds with the highest amplitude at the location determined by the vibration frequency. Perez-Gonzalez et al. [10] performed experimental measurements in 20 subjects to obtain a stiffness map of the different grasping contact areas of the human hand. The stiffness data obtained can be used as a reference for the normal stiffness of soft tissue in the different parts of the hand of healthy individuals.
Lots of researchers adopted theoretical, experimental, numerical, or finite element method to analyze the golf head, shaft, ball, etc. Tanaka et al. [11] constructed a finite element (FE) model to simulate the behaviors of a shaft during a 2D swing, and that of the impact between a golf ball and a simplified club. Pugh et al. [12] developed material tests for a two-piece golf ball and used the acquired data to produce a finite element (FE) model that is capable of predicting hysteresis during cyclic loading as well as coefficient of restitution (COR) on high speed impacts. Sandhu et al. [13] studied a model to simulate the golf drive that includes experimentally-measured inputs from actual golfers, and a three-dimensional (3D) flexible shaft model based on Rayleigh beam theory. King et al. [14] developed a wireless six degree of freedom inertial measurement units (IMU) composed of MEMS accelerometers and angular rate gyros with an integrated microprocessor and RF transceiver. This device can measure and compute the position, velocity, and orientation of the club head at the opposite end of the shaft during the entire putting stroke to support golf swing training, custom club fitting, and club design. Cheong et al. [15] showed that the major parameters, deflection, degree of torsion, frequency of vibration, and position of kick point, of a composite golf club shaft made of fiber-reinforced plastics are strongly dependent on the material properties of fibers and design pattern. Xie [16] designed golf head with a composite material by CAD software and applied the LS-DYNA software to simulate the impact of the head. Two different materials of Carbon-fiber-reinforced polymer (CFRP) and Ti are compared through the area of sweet spot zone. Xu et al. [17] investigated the biodynamic responses of the hand-arm system and employed finite element method to study the vibration of orbital sanders. They confirmed that the distributed hand responses generally varied with locations on each finger, vibration frequencies, and applied hand force. Dong et al. [18] developed a model of the handle-hand–arm system to examine the driving-point biodynamic response (DPBR) by using analytical and finite element methods. Varga et al. [19] used FEM to study fixation stability of the fractured scaphoid. The results were used to evaluate the existing and recently developed screws used to fix scaphoid fractures. Bosisio et al. [20] introduces an inverse finite-element method strategy to measure the elastic modulus and yield properties of human cortical specimens of the radial diaphysis.
Although a lot of researches have discussed the golf club or human arm separately, none of them considers the arm and golf club as a flexible dynamic system. This study emphasizes on the simulation of impact characteristic in the model combined by hand-arm and golf club mashie when it strikes at the ball.
2. 3D Hand-arm and golf club combined model
A left hand-arm and a typical golf club mashie (5-iron) are drawn in SolidWorks software according to human anatomy models and mashie’s specification as shown in Table 1. Golf head is drawn according to the parameters including inclination, elevation angle, face backward, bounce angle, etc. And the golf shaft is drawn according to the shaft length and taper angle. The assembled hand-arm and golf club mashie (HAGCM) is built up as a flexible 3D model.
Table 1Specification of golf club mashie
Rod number | Inclination | Elevation angle | Face backward | Bounce angle | Shaft length |
#5 | 23° | 61° | 5.7 mm | 3.0° | 965.2 mm |
3. Finite element analysis
The flow chart of the finite element method (FEM) includes import SolidWorks model, boundary conditions, force constraints set up, convergence test, transient (impact) analysis, etc., as shown in Fig. 1. The assembled model of hand-arm and golf club mashie includes four parts: hand-arm, golf head, golf shaft and golf grip, that each uses different materials: bone, Ti, structural steel and polyethylene, respectively, as shown in Table 2.
Table 2Material properties of the HAGCM model
Materials | Young’s modulus | Poisson’s ratio | Density g/cm3 | Damping factor |
Bone (hand-arm) | 20 GPa | 0.3 | 0.8 | 0.1 |
Titanium alloy NL (golf head) | 96 GPa | 0.36 | 4.62 | 0.00385 |
Structural steel (golf shaft) | 200 GPa | 0.3 | 7.85 | 0.008 |
Polyethylene (golf grip) | 700 MPa | 0.42 | 0.93 | 0.05 |
Fig. 1Flow chart of the FEM analysis
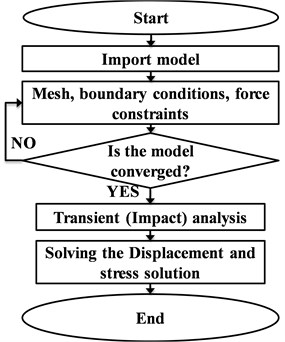
Fig. 2Meshed 3D HAGCM model
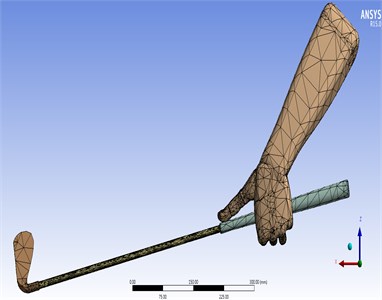
Since the club head has sharp edges and the hand-arm has complex geometry, the higher order 3-D, 10-node element SOLID187 is used to reduce the element number to the whole model (including hand-arm and golf club). There are around 25,000 elements, 43,000 nodes for the golf club, while 15,000 elements, 23,000 nodes for the hand-arm component. The meshed HAGCM assembled model is fixed as the boundary condition at the surface of the club grip as shown in Fig. 2. Finite element convergence analysis is performed for the meshed HAGCM model and is shown in Fig. 3. From the convergence plot, it can be seen that all the first four modes are converged after 38,000 elements. But for the sake of accuracy and without losing of computer performance, 40,000 elements meshed model of the HAGCM system is used for both normal and eccentric impact analysis. The deformed normal mode 18 of HAGCM model is shown in Fig. 4.
Fig. 3Convergence analysis for HAGCM model
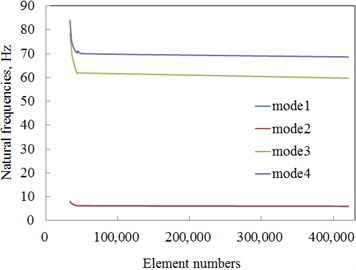
Fig. 4Deformed mode 18 of HAGCM model
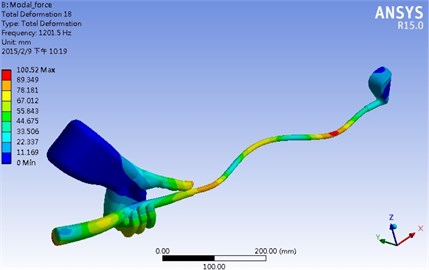
4. Result and discussion
This study is to investigate the dynamic characteristics of the HAGCM via transient (impact) analysis by FEM. Both normal and eccentric impact analysis are performed and described in the following subsections.
4.1. Normal impact analysis
The impact analysis is performed to evaluate the displacement and stress of the HAGCM system when the golf ball is hit. Transient Structural analysis of ANSYS Workbench is used to obtain the impact dynamics simulation. The elbow (proximal of the forearm) is fixed in all direction as the boundary condition. The damping coefficient is as same as the modal test and is shown in Table 2. According to Accamando (2001), the acceleration of the 45 g golf ball is around 500 m/s2 and the impact duration is about 0.01 seconds. Therefore, the impact force between the golf club and ball is 22.5 N. The location of the normal impact is at the center of mass (COM) of the club head, i.e. the head is normally hit the ball. The time responses of the deformation, principle stress and shear stress of the club head, shaft, grip and hand within 1.0 second are obtained, compared and shown in Figs. 5, 6 and 7, respectively. The descending order of the deformation amplitude is club head, shaft, grip and hand, which are all settled down within 0.5 second, as shown in Fig. 5. Two smaller deformation amplitudes of the grip and hand are zoomed in and shown in the upper block diagram of Fig. 5. The descending order of principle stress and shear stress is the club shaft, head, grip and hand, which are all settled down within 0.6 second, as shown in Figs. 6 and 7, respectively. The three smaller principle stresses on the head, grip and hand are zoomed in and shown in the upper block diagram of Figs. 6 and 7, respectively. And the maximum stress occurs at the intersection of the shaft and grip near the thumb. Figs. 6 and 7 show the stresses transmission path from the club head to the hand-arm and the stress will cause damage of those joints when golfers over practice or overuse the strength.
4.2. Eccentric impact analysis
In practice, the club head quite often hits the ball eccentrically (away from COM of the head). This section follows the same conditions described in Section 4.1 except the impact location at the tip (away from the shaft) of the head. The descending order and settling time of the deformation, principle stress and shear stress of the eccentric case are the same with that of the normal case. But the principle stress and shear stress of the eccentric case are basically larger than that of the normal case. Please refer to Fig. 8 to 10 and compare with Fig. 5 to 7, respectively.
Fig. 5Deformation of the time response of the club head, shaft, grip and hand when the club head normally hits the ball
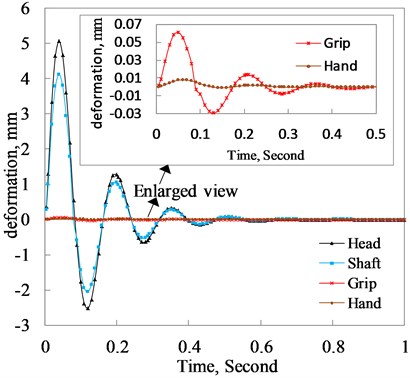
Fig. 6Principle stress of the club head, shaft, grip and hand varying with time when the club head normally hits the ball
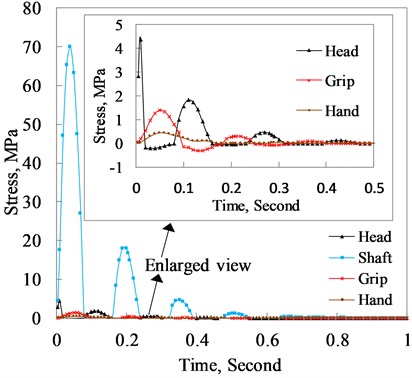
Fig. 7Shear stress of the club head, shaft, grip and hand varying with time when the club head normally hits the ball
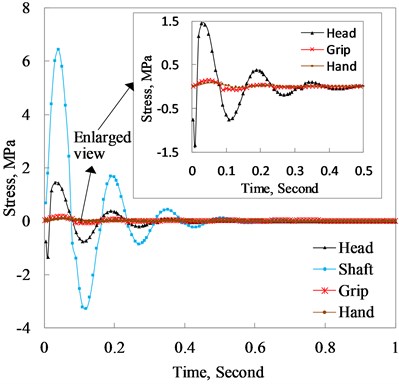
Fig. 8Deformation of the time response of the club head, shaft, grip and hand when the club head eccentrically hits the ball
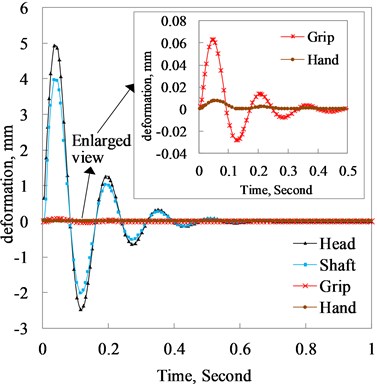
To further investigate the difference, the shear stress of the golfer hand for eccentric case is larger than that of the normal case, as shown in Fig. 11. In addition, the angular velocity of the hand-grip contacting surface for eccentric case is initially 20 times larger than that of the normal case, as shown in Fig. 12. Therefore, the golfer hand needs to exert more power to hold the shaft in order to prevent the miss shot due to rotation of the shaft for eccentric case.
Fig. 9Principle stress of the club head, shaft, grip and hand varying with time when the club head eccentrically hits the ball
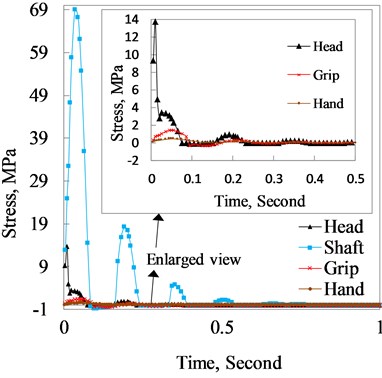
Fig. 10Shear stress of the club head, shaft, grip and hand varying with time when the club head eccentrically hits the ball
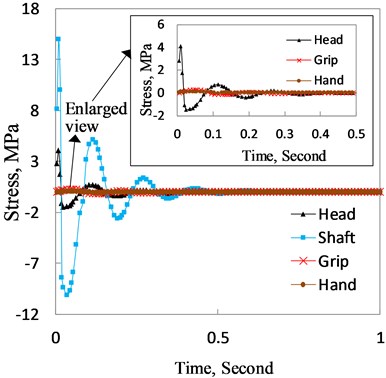
Fig. 11Shear stress response comparison of the hand between normal and eccentric hit
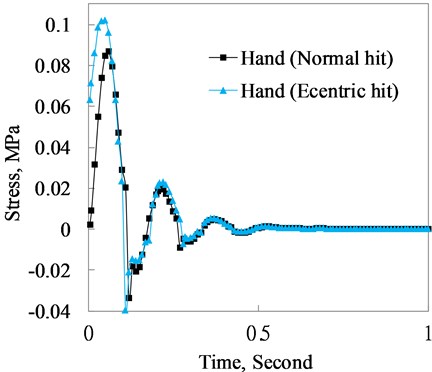
Fig. 12Angular velocity comparison of the hand-grip contacting surface between normal and eccentric hit
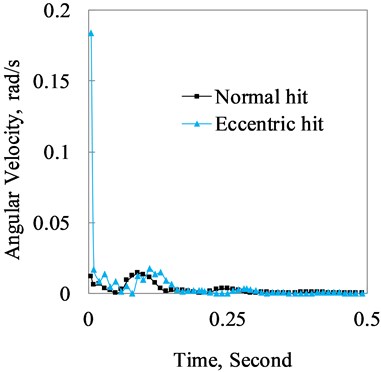
5. Conclusions
In golf swing, the various parameters such as acceleration of the club head, impact force, hit location, etc., have significant influence on the interaction between the hand-arm and golf club. Some severe cases will cause damage on fingers, wrist, elbows, etc. when golfers over practice or overuse the strength.
This paper employs finite element method to explore differences in the dynamic characteristics of HAGCM between normal or eccentric impact from the club head to the ball. The analysis of this paper is concluded as follows:
1) 3D hand-arm and golf club combined flexible model are first built to evaluate the dynamic characteristic of HAGCM system.
2) The maximum stress is occurred at the intersection of the shaft and grip near the thumb. The stress transmission path from the club head to the hand-arm is found. The stress occurred at hand-arm will eventually hurt the joints if the swing velocity of the full arm and golf club is included to produce the Coriolis force in the dynamic system.
3) The angular velocity of the hand-grip contacting surface for eccentric case is initially 20 times larger than that of the normal case. The golfer hand will exert more force to against the rotation of the golf club and it results in overused the muscle.
References
-
Archer H. A History of Golf from St. Andrews to the Masters. Amazon Digital Services, Inc., Seattle, USA, 2012.
-
Gluck G. S., Bendo J. A., Spivak J. M. The lumbar spine and low back pain in golf: a literature review of swing biomechanics and injury prevention. The Spine Journal, Vol. 8, 2008, p. 778-788.
-
Jaknowan J., Mitatha S., Vongchumyen C. Golf shaft fitting calibration system. Procedia Engineering, Vol. 32, 2012, p. 273-278.
-
Lacy Jr. T. E., Yu J., Axe J., Luczak T. The effect of driver mass and shaft length on initial golf ball launch conditions: a designed experimental study. Procedia Engineering, Vol. 34, 2012, p. 397-384.
-
Hayase S., Znuki M., Yamaguchi T. FEA and experimental investigations of principal strains and contact times during impact of golf club head and ball. Procedia Engineering, Vol. 13, 2011, p. 213-218.
-
Silva L., Marta S., Vaz J., Fernandes O., Castro M. A., Pezarat-Correia P. Trunk muscle activation during golf swing baseline and threshold. Journal of Electromyography and Kinesiology, Vol. 23, 2013, p. 1174-1182.
-
Adewusi S., Rakheja S., Marcotte P., Thomas M. Distributed vibration power absorption of the human hand-arm system in different postures coupled with vibrating handle and power tools. International Journal of Industrial Ergonomics, Vol. 43, 2013, p. 363-374.
-
Dong R. G., Rakheja S., McDowell T. W., Welcome D. E., Wu J. Z. Estimation of the biodynamic responses distributed at fingers and palm based on the total response of the hand-arm system. International Journal of Industrial Ergonomics, Vol. 40, 2010, p. 425-436.
-
Pattnaik S., Banerjee R., Kim J. Spatial resonance in a small artery excited by vibration input as a possible mechanism to cause hand-arm vascular disorders. Journal of Sound and Vibration, Vol. 331, 2012, p. 1951-1960.
-
Perez-Gonzalez A., Vergara M., Sancho-Bru J. L. Stiffness map of the grasping contact areas of the human hand. Journal of Biomechanics, Vol. 46, 2013, p. 2644-2650.
-
Tanaka K., Teranishi Y., Ujihashi S. Experimental and finite element analyses of a golf ball colliding with a simplified club during a two-dimensional swing. Procedia Engineering, Vol. 2, 2010, p. 3249-3254.
-
Pugh A., Hamilton W. R., Nash D. H., Otto S. R. Characterization of the materials in golf ball construction for use infinite element analysis. Procedia Engineering, Vol. 2, 2010, p. 3231-3236.
-
Sandhu S., Millard M., McPhee J., Brekke D. 3D dynamic modelling and simulation of a golf drive. Procedia Engineering, Vol. 2, 2010, p. 3243-3248.
-
King, K., Yoon, S. W., Perkins, N. C., Najafi, K. Wireless MEMS inertial sensor system for golf swing dynamics. Sensors and Actuators A, Vol. 141, 2008, p. 619-630.
-
Cheong S. K., Kang K. W., Jeong S. K. Evaluation of the mechanical performance of golf shafts. Engineering Failure Analysis, Vol. 13, 2008, p. 464-473.
-
Xie W. L. Design and Impact Effect Analysis of CFRP Golf Head. Master’s Dissertation, Institute of Mechatronics Engineering, National Sun Yat-Sen University, 2008.
-
Xu X. S., Welcome D. E., McDowell T. W., Wu J. Z., Wimer B., Warren C., Dong R. G. The vibration transmissibility and driving-point biodynamic response of the hand exposed to vibration normal to the palm. International Journal of Industrial Ergonomics, Vol. 41, 2011, p. 418-427.
-
Dong R. G., Welcome D. E., McDowell T.W., Wu J. Z. Analysis of handle dynamics-induced errors in hand biodynamic measurements. Journal of Sound and Vibration, Vol. 318, 2008, p. 1313-1333.
-
Varga P., Schefzig P., Unger E., Mayr W., Zysset P. K., Erhart J. Finite element based estimation of contact areas and pressures of the human scaphoid in various functional positions of the hand. Journal of Biomechanics, Vol. 46, 2013, p. 984-990.
-
Bosisio M. R., Talmant M., Skalli W., Laugier P., Mitton D. Apparent Young’s modulus of human radius using inverse finite-element method. Journal of Biomechanics, Vol. 40, 2007, p. 2022-2028.
-
Accamando E. Force of a Golf Club on a Golf Ball. The Physics Factbook, http://hypertextbook.com/facts/ 2001/Emily, 2001.