Abstract
Due to diverse applications of Lithium Nickel Manganese Cobalt (LiNixCoyMnz, NCM) composites in advanced electronics and material/alloy, the analysis of impurity composition is an important areas for evaluating its quality. In this paper, the sulfur determination in NCM composites by Inductively Coupled Plasma Optical Emission Spectroscopy (ICP-OES) was proposed. The coexisting impurities effect of Si, Fe, Mn, Mg, Ca, Ni, Cr and main matrix was investigated. Using the optimized conditions, a good linear relationship of sulfur was exhibited in the range of 0-10 mg/L (0. 9999), the standard addition recovery was 98.11-102.07 % and the RSD was 3.69 %, the levels of coexisting impurities lower than 5.0 % has no obvious interference on the determination of sulfur. This method could be served as a reliable determination of trace sulfur content in NCM composites.
1. Introduction
Driven by the social and economic progress, the newly sustainable, clean, and high-performance energy storage devices have been highly required. The civilization has been wrapped by a huge number of smart electronics gadgets, especially built with different types of sensors and communication devices [1-6]. The main classes of electrochemical energy storages are batteries, fuel cells and electrochemical capacitors et.al. Among a variety of energy storage devices, Lithium ion batteries (LIBs), as a renewable power source, have attracted wide attentions towards their long cycle life and fast charge/discharge rates [7-11]. To meet the recent demand for minimizing the size of portable electronics and electrifying transportation, it is necessary to develop efficient electrode materials for LIBs having an excellent rate capability, and cycling stability [12-14].
In a recent past, acceleration in the development of advanced technologies in the area of electronics, Lithium Nickel Manganese Cobalt (NCM) composites have been investigated in many fields of application, especially can be used as anode material for Lithium ion batteries. LiNixCoyMnz (NCM) is a ternary cathode material with high practical capacity. Many Lithium Nickel Manganese Cobalt (NCM) composites have been proved to be promising due to their low cost, high chemical stability and excellent electro catalytic properties. It is well known that NCM composites production process is of high industrial importance and some impurities could affects the properties of the product. Thus, it is important to secure the purity of the product to meet the growing demand.
The presence of sulfur in NCM composites has influences on its end functional properties in some degree. At present, the method for the determination of sulfur in NCM composites include spectrophotometry, ion chromatography, Ion selective electrode method and so on. These analytical methods also have limitations such as complex sample pretreatment processes, fluctuation value and complex detection period [15-18]. Therefore, it is of practical significance to develop a method that is simple and can meet batch sampling detection requirements. ICP-OES is a multi-element technique that allows the determination of 75 elements in the periodic table, which is simple, rapid, and achievable.
In this paper, the determination of sulfur in NCM composites by ICP-OES method was reported. Accuracy, precision and recoveries were carried out to ensure this work. Meanwhile, the influences of matrix effects were systematically clarified.
2. Methods and materials
2.1. Reagents and chemicals
Analytical reagent (AR) grade reagents and solvents were applied for all experiments. The NCM samples were supplied by Grirem Advanced Materials t Co., Ltd., China. The Ultrapure water obtained by Millipore unit (Millipore, USA) was applied for the preparation of samples. Si, Fe, Mg, Ca, Na, Zn, Cu, Zr and S standard solution of 1000 mg/L was supplied by National Institute of Metrology. In the experiment, all the gases used (H2, N2, Ar and He) were of 99.999 % purity.
2.2. Sample preparation
In a typical process, 0.1000 g sample was accurately weighed and fully dissolved in nitric acid and heated at a low temperature without other preparation. The aliquot of solution was placed in ptfe griffin beaker for several hours and then simultaneously transfer into a 100 ml flask. All samples were passed through syringe filter before injection to the test system. At specified time intervals, about 5 ml was taken from solution and analysed by ICP-OES. All Samples were prepared according to the above process.
2.3. Standard stock solution
2.3.1. Sulfur standard solution A
10 mL of sulfur standard solution (1000 mg/L) was drawn into a 100 mL volumetric flask. Sulfur standard solution of 100 mg/L was prepared as stock solution A.
2.3.2. Multi-element mixed standard solution B
10 mL of Si, Fe, Mg, Ca, Na, Zn, Cu, Zr standard solution (1000 mg/L) was drawn into a 100 mL volumetric flask, respectively. A multielement mixed stock solution B was obtained, which included Si, Fe, Mg, Ca, Na, Zn, Cu, Zr standard solution of 100 mg/L.
2.4. Standard solutions
The sulfur standard solutions between 0 and 10 mg/L were prepared by diluting the stock solution A appropriately. The substances include amount of Ni, Co, Mn and Li were added into the liquid as a base. The calculated quantification of impurity content were carried to evaluate the effectiveness of the effect of impurity influence. A slope was used to explore the recovery values during the determining of samples.
2.5. Equipment
An ICP-OES (SPECTRO ARCOS) was used for the identification and analysis of sulfur in NCM samples.
2.6. Calculation
The value of the sulfur is determined by the calculation method as bellow:
where, and (μg/mL) stand for the content of the measured component in the sample solution and blank solution; (mL) stands for the total volume of the solution; (g) stands for the quality of sample.
3. Results and discussion
3.1. Selection of optimum elemental analysis wavelength
An ICP-OES (SPECTRO ARCOS) was used in this experiment. The main operating parameters are as follows: a peristaltic pump was used with a flow rate of 2 mL/min, transmitted power 1350 W, coolant gas flow rate 0.8 L/min, auxiliary gas flow rate 0.8 L/min and nebulizer gas flow rate 0.8 L/min (Table 1). According to the optimum instrument conditions, the spectral intensity of sulfur in standard series solution, blank solution and sample solution would be measured.
The interference-free model was investigated for sulfur by recording the spectra of the sample solution and potentially interfering factor near the analytical line. Considered about the interfering factor near the analytical line and high precision for detection, the wavelength 182.034 nm was selected for this study.
Table 1Optimum operating parameters
Parameter | Value | Parameter | Value |
Transmitted power | 1350 W | Flow rate of sample uptake | 2 ml/min |
Flow rate of nebulizer gas | 0.80 L/min | Atomizer pressure | 2.8 bar |
Flow rate of auxiliary gas | 0.80 L/min | replicate readings | 3 |
Flow rate of plasma gas | 0.80 L/min | one read time | 40s |
3.2. Sample analysis
3.2.1. Linearity, LOD and LOQ
The method for sulfur analysis was validated on the basis of linearity, limit of detection (LOD), limit of quantitation (LOQ). Calibration standards of sulfur at concentration levels of 0, 1, 2, 5 and 10 mg/L were used to plot the calibration. Meantime, a good linear relationship of the proposed analytical model was obtained, as show in Fig. 1. The corresponding linear calibration curve (correlation coefficient) was 0.9999. Equation: .
Fig. 1Representative standard calibration curve of sulfur
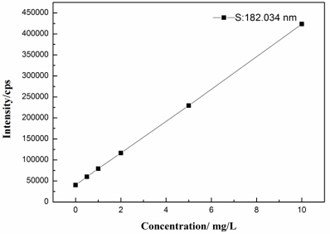
The LOD (noise ratio of 3:1) and LOQ (noise ratio of 10:1) values of this proposed method was calculated corresponding to a signal of blank samples (), as shown in Eqs. (2) and (3), respectively:
The LOD for the sulfur was 0.0031 % while the LOQ was 0.0094 %.
3.2.2. Accuracy and precision
The average (AV) sulfur present in samples was 0.0226 %. For this, the relative standard deviation (RSD) was used to estimate the repeatability and precision. RSD was 3.69 %, which was lower than 5 % for all analytes and revealed the application of ICP-OES method for sulfur analysis (Table 2).
Table 2Sulfur conten and precision of determination method (n= 7)
Sample | Content % | AV % | RSD % |
NiCoMnLi | 0.0221, 0.0232, 0.0228, 0.0231, 0.0212, 0.0234 | 0.0226 | 3.69 |
3.2.3. Analysis of recovery of standard addition
Standard recovery test was used to verify this method. The operation steps are as follows: weigh three parallel samples according to the same processing method above-mention. Then, added three different concentration levels of sulfur standard solution. It can be seen that the recovery rates of sulfur in NCM samples are between 91.88 % and 102.07 %. The specific recoveries of standard addition are shown in Table 3. These values are acceptable and effective for detecting sulfur in NCM samples.
Table 3The recovery of standard addition of sulfur in NCM samples
Sample | S Concentration (%) | Recovery rate (%) | ||
Initial | Add | Finally | ||
NiCoMnLi | 0.0226 | 0.05 | 0.0736 | 102.07 |
0.1 | 0.1194 | 96.83 | ||
0.2 | 0.206 | 91.88 |
3.3. Interference of impurity effects
According to previous studies, the impurity elements include Si, Fe, Mg, Ca, Na, Zn, Cu and Zr may be existed in the NCM materials. Atomization and excitation of sulfur may be influenced by the coexisting component and thus lead to undesired results. In this work, we carried out several experiments to verify these impurity elements interferences. The calculated standard solution B (0.1 %, 0.5 %, 1 %, 2 %) of target analyte was added to each samples and the recoveries were found in the range of 94.69 %-98.67 %, as shown in Table 4. Those results showed that impurity elements levels lower than 5 % can hardly affect the detection of sulfur in NCM samples.
Table 4Influence of Si, Fe, Mg, Ca, Na, Zn, Cu and Zr on determination of sulfur
Sample | Concentration (%) | Recovery rate (%) | ||
Initial (S) | Add (B) | Finally (S) | ||
NCM | 0.0226 | 0.1 | 0.0215 | 95.13 |
0.5 | 0.0223 | 98.67 | ||
1 | 0.0221 | 97.79 | ||
2 | 0.0214 | 94.69 |
4. Conclusions
An ICP-OES method was used for the detection and confirmation of sulfur in NCM samples. All the obtained values proves the usefulness of the method, the low values of the LOD proves the applicability of the method in samples where low amount of the sulfur is present. The average recoveries obtained by the standard addition method ranged from 84.0 % to 120.0 % with the RSD values was 3.96 %. There was no obvious interference in the determination of sulfur with coexisting impurities effect (≤ 2 %). Thus, ICP-OES as a feasible and selective analytical method can provide references for the quality evaluation of NCM composites.
References
-
X. Wang, Y.L. Ding, Y.P. Deng, and Z. Chen, “Ni‐Rich/Co‐Poor layered cathode for automotive Li‐Ion batteries: promises and challenges,” Advanced Energy Materials, Vol. 10, No. 12, p. 1903864, Mar. 2020, https://doi.org/10.1002/aenm.201903864
-
U.-H. Kim et al., “Pushing the limit of layered transition metal oxide cathodes for high-energy density rechargeable Li ion batteries,” Energy and Environmental Science, Vol. 11, No. 5, pp. 1271–1279, 2018, https://doi.org/10.1039/c8ee00227d
-
J. U. Choi, N. Voronina, Y.K. Sun, and S.T. Myung, “Recent progress and perspective of advanced high‐energy Co‐Less Ni‐Rich cathodes for Li‐Ion batteries: yesterday, today, and tomorrow,” Advanced Energy Materials, Vol. 10, No. 42, p. 2002027, Nov. 2020, https://doi.org/10.1002/aenm.202002027
-
S. N. Kwon, J. Song, and D. R. Mumm, “Effects of cathode fabrication conditions and cycling on the electrochemical performance of LiNiO2 synthesized by combustion and calcination,” Ceramics International, Vol. 37, No. 5, pp. 1543–1548, Jul. 2011, https://doi.org/10.1016/j.ceramint.2011.01.028
-
W. Zhao et al., “Optimized Al doping improves both interphase stability and bulk structural integrity of Ni-Rich NMC cathode materials,” ACS Applied Energy Materials, Vol. 3, No. 4, pp. 3369–3377, Apr. 2020, https://doi.org/10.1021/acsaem.9b02372
-
H.-H. Sun, W. Choi, J. K. Lee, I.-H. Oh, and H.-G. Jung, “Control of electrochemical properties of nickel-rich layered cathode materials for lithium-ion batteries by variation of the manganese to cobalt ratio,” Journal of Power Sources, Vol. 275, pp. 877–883, Feb. 2015, https://doi.org/10.1016/j.jpowsour.2014.11.075
-
C.-C. Pan et al., “Influences of transition metal on structural and electrochemical properties of Li[NixCoyMnz]O2 (0.6≤x≤0.8) cathode materials for lithium-ion batteries,” Transactions of Nonferrous Metals Society of China, Vol. 26, No. 5, pp. 1396–1402, May 2016, https://doi.org/10.1016/s1003-6326(16)64244-9
-
Y.-K. Sun et al., “Nanostructured high-energy cathode materials for advanced lithium batteries,” Nature Materials, Vol. 11, No. 11, pp. 942–947, Nov. 2012, https://doi.org/10.1038/nmat3435
-
Y. C. Sun, O. Y. Chu, and Z. X. Wang, “Effect of Cocontent on rate performance of LiMn0.5-xCo2xNi0.5-xO2 cathode materials for lithium-ion batteries,” Journal of The Electrochemical Society, Vol. 151, pp. 504–508, 2004.
-
M. Yoshio, H. Noguchi, J.-I. Itoh, M. Okada, and T. Mouri, “Preparation and properties of LiCoyMnxNi1−x−yO2 as a cathode for lithium-ion batteries,” Journal of Power Sources, Vol. 90, No. 2, pp. 176–181, Oct. 2000, https://doi.org/10.1016/s0378-7753(00)00407-9
-
H. Maleki Kheimeh Sari and X. Li, “Controllable cathode-electrolyte interface of Li[Ni0.8Co0.1Mn0.1]O2 for Lithium Ion batteries: a review,” Advanced Energy Materials, Vol. 9, No. 39, p. 1901597, Oct. 2019, https://doi.org/10.1002/aenm.201901597
-
Z. Wei, L. Wang, M. Zhuo, W. Ni, H. Wang, and J. Ma, “Layered tin sulfide and selenide anode materials for Li- and Na-ion batteries,” Journal of Materials Chemistry A, Vol. 6, No. 26, pp. 12185–12214, 2018, https://doi.org/10.1039/c8ta02695e
-
Q. Li, Y. Chen, J. He, F. Fu, J. Lin, and W. Zhang, “Three-dimensional VS4/graphene hierarchical architecture as high-capacity anode for lithium-ion batteries,” Journal of Alloys and Compounds, Vol. 685, pp. 294–299, Nov. 2016, https://doi.org/10.1016/j.jallcom.2016.05.293
-
W. Guo, X. Li, J. Xu, H. K. Liu, J. Ma, and S. X. Dou, “Growth of highly nitrogen-doped amorphous carbon for lithium-ion battery anode,” Electrochimica Acta, Vol. 188, pp. 414–420, Jan. 2016, https://doi.org/10.1016/j.electacta.2015.12.045
-
X. N. Zhang, “Trace levels determination of fluorine, chlorine, sulfate and nitric acid in water by ion chromatography,” Shanxi Chemical Industry, Vol. 37, No. 4, pp. 47–49, 2017, https://doi.org/10.16525/j.cnki.cn14-1109/tq.2017.04.15
-
X. F. Yao and J. X. Li, “Improvement of quality method for determination of sulfate content in soluble salt,” Shanxi Architecture, Vol. 44, No. 23, pp. 49–50, 2018, https://doi.org/10.13719/j.cnki.cn14-1279/tu.2018.23.027
-
Wu Kai-Hong, Liang Hua-Mei, He Zhi-He, Zhang Ying-Jiao, Guangdong Brunp Recycling Technology Co., and Ltd., “Determining content of sulfate radical in NixCoyMn1-x-y (OH) 2 by ICP-AES,” Battery Bimonthly, No. 2, pp. 117–119, 2016.
-
Liang Ming-Xia, “Determination of chlorine and bromine in printed circuit boards by ICP-OES,” Guangzhou Chemical Industry, Vol. 39, No. 23, pp. 117–118, 2011.
About this article
The authors acknowledge the financial support by National test and evaluation platform for advanced materials- Primary center (TC170A5SU/1) and National test and evaluation platform for advanced materials – Nonferrous metals center (TC190H3ZW/2).