Abstract
This work focuses on the fabrication and testing of highly conductive polymer electrodes to measure the biomedical signals known as an Electrocardiogram (ECG). When doing this, optimal skin contact is considered. Align Multiwalled Carbon Nanotubes (AMWCNT), Graphene Nanoplates (GNPs), and Polydimethylsiloxane (PDMS) were used to fabricate the electrodes for continuous ECG monitoring. Sonication and magnetic stirring were used to disperse the mixture. The conductance of the PDMS/GNPs/AMWCNT electrodes were measured using a digital multimeter and were compared with that of standard silver-silver chloride (Ag/AgCl) electrodes. The current study showed that real-time monitoring achieved by an internet of things (IoT) systems with microcontroller.
1. Introduction
Today, studies on wearable technologies which are the subject of many classical studies, represent a growing field and many researchers are trying to develop perfectly suitable devices. The development of a wearable electrode is critical to achieving that purpose because the current data monitoring over the specified period is related to the quality of data obtained from a source. Electrodes are known as the fundamental components that affect the quality of the signal obtained from the source. These electrodes allow us to reach electrical information by transmitting the current. It can also be part of flexible sensors in complicated cases, or sometimes the sensing element itself. While the human body has a soft and curved structure, the electrode materials, which are produced via traditional methods such as thin film deposition or another method for classic production are hard, brittle, and flat, making it difficult to detect instant, localized biological measurements directly from human skin [1-4]. This system is not only for humans, but can also be used in animal experiments. Kviesulaitis et al. took ECG measurements on sheep for 24 hours in their experiment. In their study, they said that the electrodes caused skin irritations, and this problem was prevented with the electrodes made in this study [5].
Therefore, the future development of electrodes needs the alteration from solid-state electronics to a flexible material [6]. Stretchable, flexible, biocompatible electronic materials are of great interest for use in such existing applications. Various nano-composite materials which are combined with nanomaterials and elastic polymers have been the subject of such flexible electronic materials studies since 2000 [7-11].
Particularly in this area, polymers offer new possibilities for advances in electrode material research. Various nanomaterials are added to polymers to gain new functions when they become a whole with the properties inherent in polymers. Moreover, this incorporation can also result in high-performance porous materials for use in many fields [9]. Polydimethylsiloxane, polyurethane (PU), polyimide (PI), polystyrene (PS), polycarbonate (PC), polylactic acid (PLA), and polyvinyl chloride (PVC) are various polymers that have been used in studies so far to create polymer composites. [12, 13]. Among them, PDMS has been focused on in scientific research as it offers a wide range of applications to researchers, and it is elastic, biocompatible, and easily structured properties. Most importantly, they are widely used in electronic applications because they produce smart materials as a result of nano-fillers added to these elastomeric polymers [14, 15]. The most common nanofiller material added to polymers is the carbon nanotube (CNT), which can be found in two forms: multi-walled carbon nanotube (MWCNT) and single-walled carbon nanotube (SWCNT). The carbon nanotube network provides polymers with high electrical conductivity and a low percolation threshold. Carbon nanotubes are ideal nanofiller materials because of their positive contribution to thermal conductivity [16].
Jung et al. produced a carbon nanotube (CNT) polydimethylsiloxane (PDMS) composite-based dry electrocardiogram (ECG) electrode that can be easily connected to conventional ECG devices and demonstrated its long-term wearable monitoring capability, resistance to movement and sweating [2].
The standard silver-silver chloride (Ag/AgCl), dry and capacitive electrodes that we commonly use in the measurement of ECG signals, use conductive gel to maintain better electrical contact with the skin and therefore provide better results [1]. However, the need for conductive gel may cause skin problems such as irritation or allergic reactions and it is unpreventable with nanocomposite electrodes. Therefore, the disadvantages such as ECG dry electrodes, skin problems, redness, failure in long-term measurements, or slower and more sensitive data acquisition. There has been a trend towards ECG flexible electrodes in healthcare and other industrial areas.
ECG measurement as a phenomenon is explained as electric potential and occurs as a result of the potential difference between any pair of electrodes. The conversion of electrical potential signals by the electrocardiograph is known as the electrocardiogram (ECG) and each cardiac cycle has a P wave, QRS complex, and T wave as shown in Fig. 1 [17].
Fig. 1The image of the AMWCNT/GP/PDMS composite structure and the waves of scientifically accepted ECG signals are shown in the graph [18]
![The image of the AMWCNT/GP/PDMS composite structure and the waves of scientifically accepted ECG signals are shown in the graph [18]](https://static-01.extrica.com/articles/23201/23201-img1.jpg)
Due to the PDMS and CNT properties mentioned above, for example, it is seen that materials that are flexible, biocompatible, physically suitable for the human body, can be shaped as desired and also have high electrical and thermal conductivity are chosen in the production of ECG electrodes due to these properties. This paper demonstrates the usage of nanocomposite PDMS/CNT/GNP electrodes to measure electrical signals produced by human heart with each beat.
2. Experimental
2.1. Materials
The materials used for developing ECG electrodes consist of AMWCNT and GNP mixed with different weight ratios (5 %, 7 %, 9 %) in the PDMS matrix, which is given in Table 1.
Table 1AMWCNT/GP/PDMS composite mixture ratios CNT/GNP dispersion in PDMS
Groups | Percent | Ratio | Poly-dimethyl siloxane (gr) | Align Multi-walled carbon nanotubes (gr) | Graphene (gr) |
1. | 5 % | 6:1 | 10 | 0,42 | 0,08 |
2. | 7 % | 6:1 | 10 | 0,6 | 0,1 |
3. | 9 % | 6:1 | 10 | 0,77 | 0,13 |
AMWCNTs were obtained from Nanografi (Ankara, TURKEY), which has > 95 % purity. Nanotubes in terms of diameters 10-20 nm and length is between 1-2 µm. In addition, it has a 2.5 g/mL density at room temperature. GNPs were also purchased from Nanografi, it has a purity of over 99.9 %. GNP’s thickness is around 5 nm and its diameter is 18 nm. Sylgard 184 Silicone Elastomer (PDMS) is the polymer used because of its impressive characteristics like biocompatibility, non-toxicity, and flexibility and purchased from Dow Corning, Michigan, USA.
Fig. 2A schematic illustration of four different modifications for CNTs or GNPs interacting with polymer matrix [19]
![A schematic illustration of four different modifications for CNTs or GNPs interacting with polymer matrix [19]](https://static-01.extrica.com/articles/23201/23201-img2.jpg)
The ideal dispersion of material inside the composite is related to the application, and how they were dispersed showed Fig. 3 and isopropyl alcohol (IPA) was used for dispersing CNTs and GNPs in PDMS. Even though, CNT-GNP nanocomposites might not be as tough or solid as a reinforced composite such as carbon fiber laminates. However, the multifunctionality of CNTs and graphene is still becoming game-changers. In addition to the various challenges of dispersion, viscosity control, sizing, and alignment of these materials.
2.2. Fabrication of ECG electrodes
Multi-walled carbon nanotubes and graphene nanoplatelets were weighed the desired ratio. Then, 3 different percentages of AMWCNT/GNP according to weight of PDMS were added to the beakers. These percentages are; 5 %, 7 %, 9 %. The calculations were made considering the 6:1 ratio. According to the ratios and concentration, the mixtures were named as PDMS5, PDMS7, and PDMS9. AMWCNT and GNP were first dispersed in 50 ml of isopropyl alcohol (IPA) using magnetic stirrer for 10 minutes. The solution was kept in an ultrasonic bath at 40 kHz for 30 minutes. After uniform dispersing was obtained, of the PDMS A form with 10 gr was mixed with the solution followed by mechanical stirring for 20 minutes. The composite solution was then placed on a hot plate with magnetic stirring for exsiccating of IPA and overnight waited at 100 degrees. After that, a PDMS B form (crosslinking agent) was added in 10:1 into the composite and degassed completely in a can be used vacuum. After the exsiccation of air in the mixture, nanocomposites were immediately molded on already prepared molds, these steps are shown in more detail in the following Fig. 3. AMWCNT/GNP/PDMS hybrid nanocomposites were cured in prepared steel molds at 120 °C for 30 minutes.
Fig. 3Schematics of composite fabrication for ECG electrodes
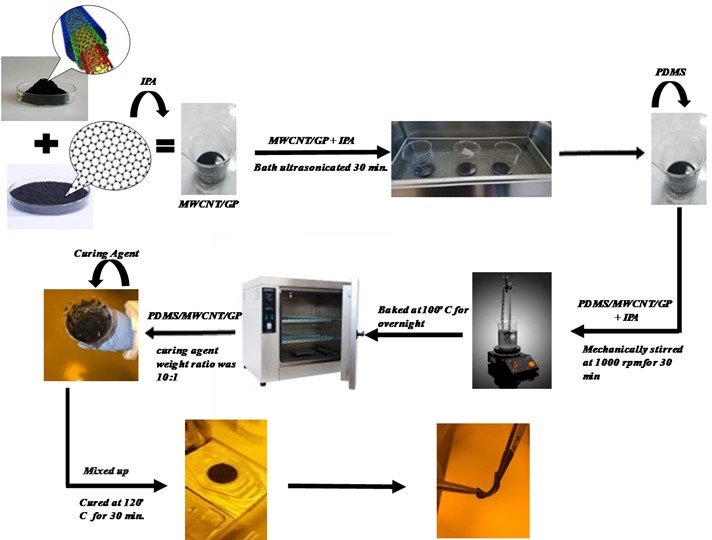
3. Results and discussion
3.1. Conductivity
PDMS5, PDMS7 and, PDMS9 samples were cut into 1×1 cm2 rectangular pieces. The thickness of each hybrid nanocomposite was measured with a digital micrometer. Thickness measurements of each hybrid sample were made from 3 different points previously determined on the surface and made averages. Thickness measurements of each hybrid sample are shown in Table 2.
Table 2Thickness measurements for dry electrodes.
Samples | Thickness (mm) | |||
1st measurement | 2nd measurement | 3rd measurement | Average | |
6:1 (5 %) | 16,2 | 16,3 | 16 | 16,1 |
6:1 (7 %) | 15,6 | 15,2 | 16 | 15,6 |
6:1 (9 %) | 16,5 | 16 | 16,1 | 16,2 |
The electrical conductivity measurements of the samples were calculated from the plate resistance () values obtained by using flux, and the siemens measurement (S/m) unit was used. Here, firstly, as a result of the resistance (Ohm.m) values obtained, the transition to resistivity () is made by using Eq. (1). Then, Eq. (2) was applied to the resistivity values found:
The correlation and variation between nanocomposite electrodes are shown in the Fig. 4. It was observed that the electrical conductivity of the nanocomposite structure increased as the weight of the carbon nanotube and graphene placed in the mixture increased. Although this change is not linear, it increases exponentially in the percolation threshold region. That is quite low for aligned carbon nanotubes graphene nanoplates nanocomposites.
Fig. 4Conductivity of the produced electrodes is in the form of siemens
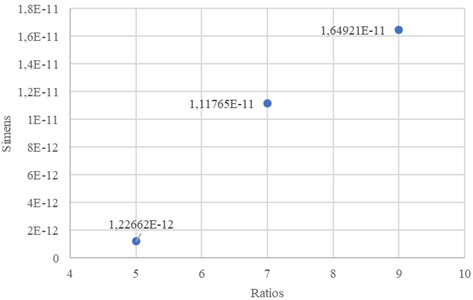
3.2. Fourier transform infrared spectroscopy (FTIR)
FTIR Spectrum100 (Perkin Elmer, Waltham, MA) was used. The frequency range was 650-4000 cm-1 wavenumber in the transmittance mode and FTIR is one of the important characterization techniques for identifying functional groups in AMWCNT/GNP/PDMS.
Structural properties of the different ratios at AMWCNT/GNP/PDMS were measured by FTIR spectroscopy in the frequency range of 650-4000 cm-1 (Fig. 5). The FTIR spectrum of AMWCNT/GNP/PDMS was characterized by a symmetrical stretching vibration of -CH3 at 2962 cm−1, deformation vibration of -CH3 in Si-CH3 at 1257 cm-1, Si-O-Si asymmetrical deformation at 1005 cm-1, C-O-S stretching at 911 cm-1. The absorption band appeared at 863,789 cm-1, respectively, representing -CH3 symmetrical rocking. The peaks correspond to Si-C asymmetrical stretching, Si-C symmetrical stretching, and Si-CH3 symmetrical rocking in nanocomposite were observed at 843,758, and 687 cm-1, respectively. As can be seen from the results obtained, mixing AMWCNT/GNP to PDMS at increasing rates changed the intensities of the PDMS’s bands and led to the emergence of new bands in the spectrum that would be attributed to AMWCNT/GNP. This indicates that there is an interaction between PDMS and AMWCNT/GNP.
3.3. ECG measurement setup
From the results obtained for conductivity, it can be said that the nanocomposite of PDMS with align-MWCNTs proved to be a better choice and preferable for ECG monitoring. The ECG measurement system was obtained through an Arduino Uno board coupling with an AD8232 cardiac sensor board. Therefore, the cardiac cycles of producing flexible electrodes have been obtained using Arduino software.
An ECG measurement was taken from the chest of a subject for 15 seconds using AMWCNT/GNP/PDMS electrode and as a control Ag/AgCl dry electrode and compared.
Fig. 5FTIR spectra of AMWCNT/GNP/PDMS at differential ratios
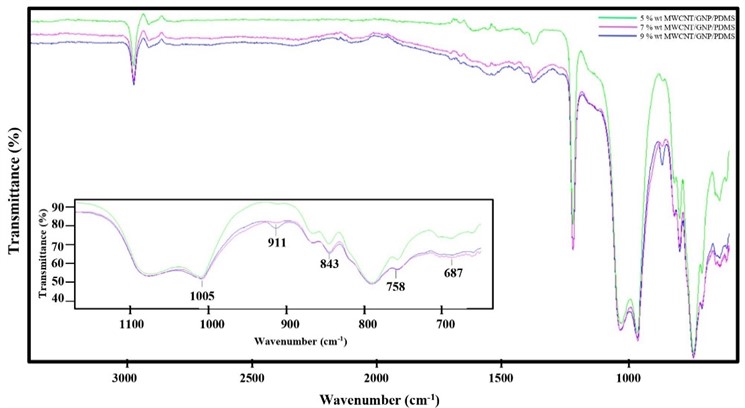
3.4. Heart cycles with Arduino uno board as a microcontroller
The ECG signal quality, while the body is fixed, was then analyzed and compared between the wet Ag/AgC1 electrode and the printed dry ECG electrodes. The cardiac cycles of produce flexible electrodes have been obtained by used software in Arduino and have been shown that comparative with dry-electrode in Fig. 6. When the ECG signal of AMWCNT/GNP/PDMS and Ag/AgCl electrodes are compared at the same time, it seems that there is no significant difference between the two signals. When the peaks are examined considering the waves occurring in the cardiac cycle, it is seen that P, QRS and T waves can be clearly selected. The waveform of the ECG signal from the AMWCNT/GNP/PDMS electrode almost exactly matches that from the Ag/AgCl electrode.
Fig. 6Schematics of MWCNT/GNP/PDMS heart cycles in ECG electrodes
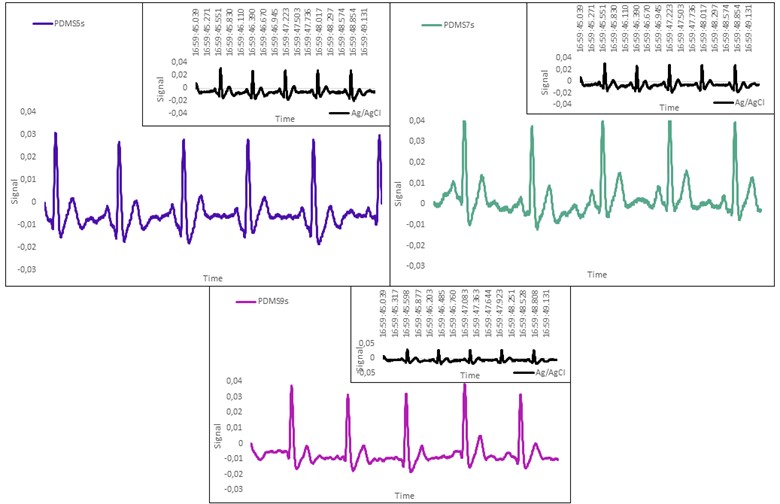
In line with the data, all cardiac cycle waves are seen in the ECG measurements taken. It is seen that the PDMS9 (Fig. 6) electrodes transmit the cleanest signal. However, studies on these electrodes should continue in the future and the most accurate result can be achieved by using signal processing. The data of all cardiac cycle waves should be examined separately as a long term.
4. Conclusions
Three dry nanocomposite electrodes with varying mixing ratios were created in this study and compared to wet commercial Ag/AgCl electrodes. A sensitive ECG electrode made of AMWCNT, GNP, and PDMS was created. In the ECG frequency range, the composite material's average conductance was 1 S. The conductivity was rarely impacted by the electrode thickness.
PDMS/MWCNT, dry Ag, and Ag/AgCl electrodes were used to take ECG readings from a participant who was at rest for 15 seconds (on the chest). It was determined that the AMWCNT/GNP/PDMS electrodes performed well when dry Ag electrodes and regular Ag/AgCl electrodes were compared for quality. The results show great promise for using PDMS/MWCNT composites for biopotential sensing, with a higher SNR than earlier research.
In order to create flexible electrodes, PDMS, GNPs, and Align-MWCNT were used. Three electrodes were created, ranging in MWCNT content from 5, 7 and 9 weight percent in PDMS. The results from 9 wt%, which comprises 8:1 AMWCNT: GNP and is comparable to ordinary electrodes, were the best. An IoT-based ECG tracking system was used to track the ECG signals in real-time. Because the electrodes were biocompatible and showed no evidence of skin irritation or allergy even after five days, they were ideal for long-term and distant monitoring.
References
-
T. H. Park, J.H. Kim, and S. Seo, “Facile and rapid method for fabricating liquid metal electrodes with highly precise patterns via one‐step coating,” Advanced Functional Materials, Vol. 30, No. 35, p. 2003694, Aug. 2020, https://doi.org/10.1002/adfm.202003694
-
Ha-Chul Jung et al., “CNT/PDMS composite flexible dry electrodes for long-term ECG monitoring,” IEEE Transactions on Biomedical Engineering, Vol. 59, No. 5, pp. 1472–1479, May 2012, https://doi.org/10.1109/tbme.2012.2190288
-
Z. Ye, Q. Li, R. Zhang, P. Zhang, and L. Gui, “Fabrication of a thin PDMS film with complex liquid metal electrodes embedded and its application as skin sensors,” RSC Advances, Vol. 12, No. 14, pp. 8290–8299, Mar. 2022, https://doi.org/10.1039/d1ra09394k
-
W. Xi et al., “Soft tubular microfluidics for 2D and 3D applications,” Proceedings of the National Academy of Sciences, Vol. 114, No. 40, pp. 10590–10595, Oct. 2017, https://doi.org/10.1073/pnas.1712195114
-
V. Kviesulaitis, T. Kazakevičius, V. Zabiela, and A. Puodžiukynas, “An approach to 24-hour electrocardiogram monitoring in sheep experimental model,” Journal of Measurements in Engineering, Vol. 6, No. 2, pp. 119–125, Jun. 2018, https://doi.org/10.21595/jme.2018.20028
-
S. Zhang et al., “Hydrogel‐enabled transfer‐printing of conducting polymer films for soft organic bioelectronics,” Advanced Functional Materials, Vol. 30, No. 6, p. 1906016, Feb. 2020, https://doi.org/10.1002/adfm.201906016
-
F. Jiang, G.-B. Lee, Y.-C. Tai, and C.-M. Ho, “A flexible micromachine-based shear-stress sensor array and its application to separation-point detection,” Sensors and Actuators A: Physical, Vol. 79, No. 3, pp. 194–203, Feb. 2000, https://doi.org/10.1016/s0924-4247(99)00277-0
-
J. H. Kim et al., “Simple and cost-effective method of highly conductive and elastic carbon nanotube/polydimethylsiloxane composite for wearable electronics,” Scientific Reports, Vol. 8, No. 1, pp. 1–11, Jan. 2018, https://doi.org/10.1038/s41598-017-18209-w
-
T. R. Michel et al., “Evaluation of porous polydimethylsiloxane/carbon nanotubes (PDMS/CNTs) nanocomposites as piezoresistive sensor materials,” Microsystem Technologies, Vol. 26, No. 4, pp. 1101–1112, Apr. 2020, https://doi.org/10.1007/s00542-019-04636-4
-
Y. Jung, K. K. Jung, D. H. Kim, D. H. Kwak, and J. S. Ko, “Linearly sensitive and flexible pressure sensor based on porous carbon nanotube/polydimethylsiloxane composite structure,” Polymers, Vol. 12, No. 7, p. 1499, Jul. 2020, https://doi.org/10.3390/polym12071499
-
O. Kanoun, A. Bouhamed, R. Ramalingame, J. R. Bautista-Quijano, D. Rajendran, and A. Al-Hamry, “Review on conductive polymer/CNTs nanocomposites based flexible and stretchable strain and pressure sensors,” Sensors, Vol. 21, No. 2, p. 341, Jan. 2021, https://doi.org/10.3390/s21020341
-
E. Aram and S. Mehdipour-Ataei, “A review on the micro – and nanoporous polymeric foams: Preparation and properties,” International Journal of Polymeric Materials and Polymeric Biomaterials, Vol. 65, No. 7, pp. 358–375, May 2016, https://doi.org/10.1080/00914037.2015.1129948
-
M. Sauceau, J. Fages, A. Common, C. Nikitine, and E. Rodier, “New challenges in polymer foaming: A review of extrusion processes assisted by supercritical carbon dioxide,” Progress in Polymer Science, Vol. 36, No. 6, pp. 749–766, Jun. 2011, https://doi.org/10.1016/j.progpolymsci.2010.12.004
-
R. Ramalingame, A. Lakshmanan, F. Müller, U. Thomas, and O. Kanoun, “Highly sensitive capacitive pressure sensors for robotic applications based on carbon nanotubes and PDMS polymer nanocomposite,” Journal of Sensors and Sensor Systems, Vol. 8, No. 1, pp. 87–94, Feb. 2019, https://doi.org/10.5194/jsss-8-87-2019
-
A. Subramaniam and S. Sethuraman, “Biomedical applications of nondegradable polymers,” Natural and Synthetic Biomedical Polymers, pp. 301–308, 2014, https://doi.org/10.1016/b978-0-12-396983-5.00019-3
-
A. Kausar, “Polydimethylsiloxane-based nanocomposite: present research scenario and emergent future trends,” Polymer-Plastics Technology and Materials, Vol. 59, No. 11, pp. 1148–1166, Jul. 2020, https://doi.org/10.1080/25740881.2020.1719149
-
A. Galli, R. J. H. Montree, S. Que, E. Peri, and R. Vullings, “An overview of the sensors for heart rate monitoring used in extramural applications,” Sensors, Vol. 22, No. 11, p. 4035, May 2022, https://doi.org/10.3390/s22114035
-
J. Gordon Betts et al., Anatomy and Physiology. Houston, Texas: OpenStax, 2013.
-
I. A. Kinloch, J. Suhr, J. Lou, R. J. Young, and P. M. Ajayan, “Composites with carbon nanotubes and graphene: An outlook,” Science, Vol. 362, No. 6414, pp. 547–553, Nov. 2018, https://doi.org/10.1126/science.aat7439
About this article
This research was supported by Marmara University Scientific Research Project Coordination Unit (BAPKO) as project number FYL-2022-10500.
The datasets generated during and/or analyzed during the current study are available from the corresponding author on reasonable request.
The authors declare that they have no conflict of interest.