Abstract
To explore the vibration characteristics and seismic performance of historical buildings in Tianjin, we conducted on-site vibration testing under ambient vibration on three historical buildings with brick-wood structures in Tianjin. Specifically, we delved into the vibration characteristics and seismic performance of historic buildings with brick-wood structures by establishing the vibration analysis model, performing the theoretical calculations, and conducting seismic performance analysis. The results reveal that 1) the vibration frequencies of historical buildings with brick-wood structures are low, mainly concentrated in 2.0-8.0 Hz, which conforms to the vibration range of general buildings; 2) based on vibration analysis, it is concluded that the structural integrity of these buildings is good and there are no obvious structural defects; 3) the layout and storey height of historical buildings have great impacts on the vibration characteristics and seismic performance. 4) the comprehensive seismic capacity index of three buildings ranges from 0.91 to 1.75. The results of dynamic analysis under ambient vibration are consistent with those of theoretical analysis, providing a basis for preserving and reinforcing historical buildings.
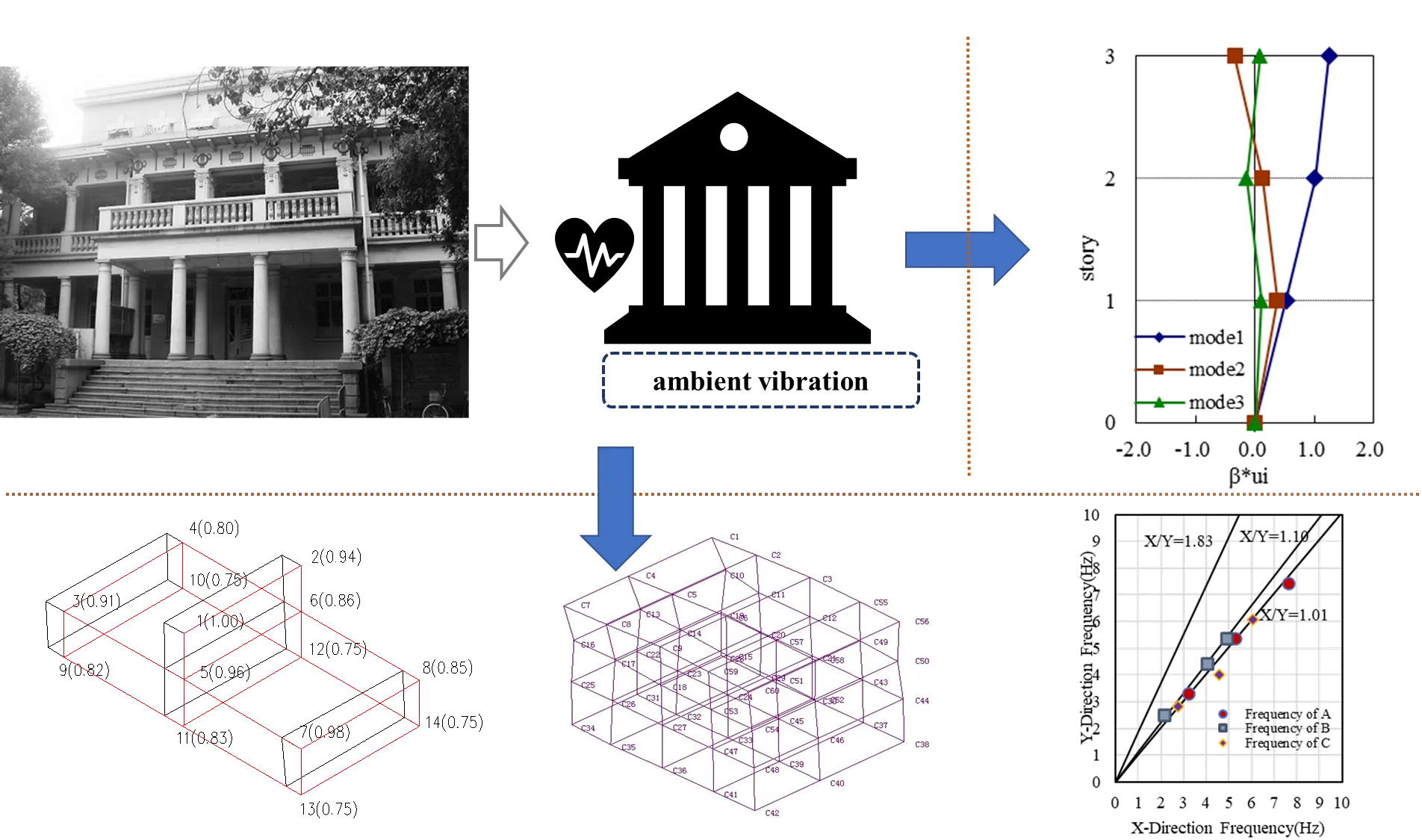
Highlights
- The experiment of historical buildings under environmental vibration was completed.
- The results of theoretical analysis reveal that vibration experiment can effectively evaluate the structural characteristics of historical buildings.
- This study provides a basis for the preservation of historical buildings in Tianjin.
1. Introduction
Historical buildings witness the development of human civilization and the progress of science and technology. Therefore, the research on safety assessment and the formulation of conservation models for these buildings has received great attention from various countries [1-4]. China’s historical buildings are an essential part of Chinese culture and valuable assets of the people of China and the world. Tianjin is a renowned city characterized by unique architectural features and historical culture. In 2005, Tianjin promulgated and implemented the Regulations on the Protection of Buildings with Historic Features in Tianjin. As of 2014, it had accredited 877 buildings with historical features [5], of which historic buildings with brick-wood structures occupied a large proportion [6]. Building with historical features is a legal term coined by Tianjin to protect historic buildings. Since the end of the 20th century, Tianjin has been preserving buildings with historical features on a large scale, making some achievements.
Buildings with historical features integrate history and science and reflect the development of science and technology. They are of great heritage and aesthetic value. In recent years, numerous experts and scholars have researched and studied historical buildings from various perspectives, compiled a host of valuable technical data, and repaired some historical buildings with structural defects, providing strong technical support for the preservation of these buildings [3, 7, 8]. However, regarding the preservation of historical buildings, scholars have long been attaching importance to architectural art, ignoring structural performance. It lacks research achievements on the safety and seismic resistance of historical buildings. However, most of these buildings, especially those built in the early years, severely deteriorate safety performance. Due to the low strength of materials and the limitations of theoretical cognition, the overall seismic performance of the structure of these buildings is poor [9, 10]. Currently, there are many cases of damage to historical buildings caused by traffic and earthquakes at home and abroad [11-14]. China is an earthquake-prone country, with many historical buildings located in highly seismic regions. Hence, the study of vibration characteristics and the seismic performance is not only of great significance to the preservation of historical buildings, but also provides references for the reinforcement and maintenance of these buildings.
Buildings with historical features in Tianjin are diverse in form and large in number, with the building materials, structural and construction forms significantly different from those of modern buildings. Generally speaking, these buildings were constructed within a concentrated period in a cluster of locations, with complex architectural styles and unique building materials [15]. After the inspection, investigation, and study of the historical buildings in Tianjin, we found that these buildings mainly adopt brick-wood structures, wood structures, and brick and concrete structures [16]. Due to the uneven seismic performance of brick-wood buildings with historical features, it is difficult to assess their seismic performance accurately. In terms of safety and seismic performance, although some seismic measures of most buildings with historical features do not satisfy the requirements of current seismic specifications and seismic identification standards, they show good seismic performance in many strong earthquakes. Therefore, the study of vibration characteristics and seismic performance of brick-wood buildings with historical features is not only beneficial to the protection of historical features of buildings, but also provides a reference for the design of modern buildings.
In this study, we selected three buildings with historical features in Tianjin for vibration testing and theoretical calculation analysis, explored their dynamic characteristics, such as vibration frequencies and mode shapes, and verified their seismic performance using the calculation data. Additionally, we clarified the characteristics of these historical features and elaborated on the reasons for not suffering from damage caused by strong earthquakes, thus laying the foundation for later in-depth research. The vibration characteristics and seismic performance of historical buildings are different from those of general buildings, and the traditional theoretical analysis methods cannot reasonably evaluate their safety. This study combines vibration testing and theoretical analysis results to reasonably evaluate its structural characteristics.
2. Overview of three historical buildings
In this study, we explored three historical buildings with brick-wood structures in Tianjin. Building A is the former residence of Duan Qirui (a Chinese warlord and politician), Building B is Jingyuan (the former residence of Puyi, the last emperor of China), and Building C is a small western-style building located at No. 121 Machang Road. We figured out their vibration characteristics and evaluated their safety performance through testing and computational analysis under ambient vibration. The details of three historical buildings are shown in Table 1. The position of sensors in the building is shown in Fig. 1.
Table 1Details of 3 historical buildings.
Building name | Year of construction | Number of floors | Plan size | Area |
Building A | 1920 | Three floors (a basement) | 25.9m×26.2 m | 2430 m2 |
Building B | 1921 | Two floors (some parts of the building have three floors) | 36.5m×20.0 m | 3360 m2 |
Building C | 1905 | Three floors (a basement) | 16.46 m×14.91 m | 576 m2 |
Located in the Heping District of Tianjin, Building A was built in 1920. It is a historical building with a classical European style. It has a building area of about 2,430 m2. It is a three-storey building with brick-wood structures and a semi-basement. Its planar structure is basically symmetrical, 25.9 m in the north-south direction ( direction) and 26.2 m in the east-west direction ( direction); while its vertical structure is unevenly distributed, in which the basement is 2.74 m high, the first floor is 4.74 m high, and the second and third floors are 5.18 m high. Due to the long history of natural disasters such as earthquakes, and man-made sabotage such as wars, Building A has issues such as the decrease in safety and the gradual deterioration of architectural functions. The architectural appearance and ground floor plan of Building A are shown in Fig. 1.
Fig. 1Building A
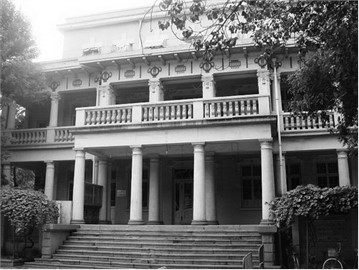
a) Architectural appearance of building A
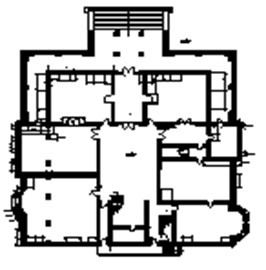
b) Ground floor plan
Building B is located in Heping District, Tianjin City. Built in 1921, it was designated as a historical building with special protection level by Tianjin Municipal People’s Government, with the renovation project completed in 2007. The main body is a Spanish-style two-storey (some parts of the building are three-storey) building with brick-wood structures. The first floor is 4.50 m high, the second floor is 3.93 m high, and the third floor is 4.03 m high. The building is long in the north-south direction ( direction), about 36.5 m, and short in the east-west direction ( direction), about 20.0 m. The planar structure is rectangular, while the horizontal and longitudinal layout is regular. The architectural appearance and ground floor plan are shown in Fig. 2.
Fig. 2Building B
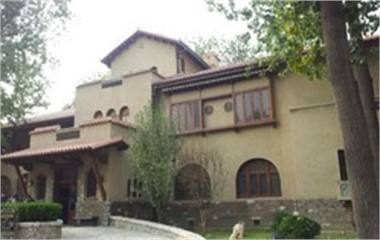
a) Architectural appearance of building B
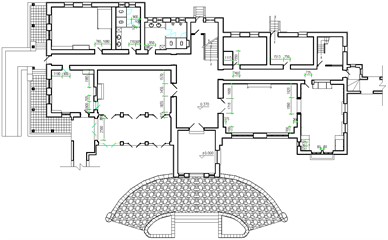
b) Ground floor plan
Building C is located at No. 121 Machang Road, south of downtown Tianjin City. It is a three-storey building with brick-wood structures and a basement. It is 16.46 m long in the east-west direction ( direction) and 14.91 m long in the north-south direction ( direction). The planar and vertical structures are symmetrical. The first floor is 3.6 m high, and the other floors are 3.3 m high. Fig. 3 shows the architectural appearance and ground floor plan of Building C.
3. Vibration testing
3.1. Outline
The understanding of the dynamic characteristics of the structure is an important premise for us to figure out the distribution of mass and stiffness, perform safety evaluation and seismic design, solve engineering vibration issues, and diagnose accumulated damage. Italy and other European countries attach great importance to the conservation of historical buildings, and they have accumulated rich achievements in the analysis of the dynamic characteristics of historical buildings [17-19]. The domestic research on dynamic characteristics of historical buildings started late, especially the use of on-site testing techniques. With the development of computer and sensing technology, more and more researchers have gradually employed vibration testing to analyze the dynamic characteristics, such as natural frequency, mode shape, and damping coefficient, of historical buildings [20, 21]. Besides, based on vibration testing and theoretical analysis, they conducted in-depth research on the stiffness and seismic performance of the structure using modal analysis, inversion calculation, and analog simulation [22, 23].
Fig. 3Building C
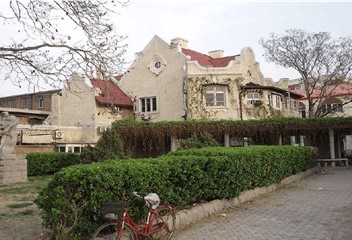
a) Architectural appearance of building C
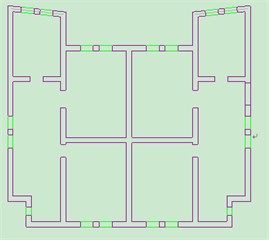
b) Ground floor plan
The dynamic characteristics of historical buildings are the intrinsic parameters of the structure, including the natural vibration frequency, damping coefficient, and mode shape of the structure. Given the results of testing under ambient vibration can reflect the dynamic characteristics of three brick-wood buildings with historical features in this study, we employed the pulsation test to carry out vibration testing and data analysis.
3.2. Test instruments and contents
The analysis results indicate that the vibration of three historical buildings is mainly at low frequency, with low vibration velocity. According to the structural characteristics and sensor test range, we adopted the ultra-low frequency vibrometer and modal analysis system for vibration testing. The ultra-low frequency vibrometer is a high-performance and low-frequency vibration sensor. It is a moving-coil reciprocating vibration picker. The main technical specifications of the sensor are shown in Table 2. We employed the DHMA experimental modal analysis system produced by Jiangsu Donghua Testing to measure and analyze vibration. The system consists of a controllable excitation system, sensor, data acquisition system, experimental mode analysis software, etc. By analyzing the self-power spectrum, cross-power spectrum, correlation function, and transfer rate obtained from the sample data, DHMA mode experimental system can accurately obtain the modal parameters (natural frequency, mode shape, damping ratio) of the structure under test and display the 3D animation of the mode shape of the structure under test.
Table 2Main technical indicators of the sensor
Sensitivity ((V·s2)/m or V·s/m) | Gear indicator | ||||
1 | 2 | 3 | 4 | ||
Acceleration | Low speed | Medium speed | High speed | ||
0.3 | 23 | 2.4 | 0.8 | ||
Max. range | Acceleration (m/s2, 0-p) | 20 | – | – | – |
Speed (m/s, 0-p) | – | 0 .125 | 0.3 | 0.6 | |
Displacement (mm, 0-p) | – | 20 | 200 | 500 |
Since the vibration signals of historical buildings under study are mainly low-frequency, we set the sampling frequency to 2.56 times the analysis frequency and used a low-pass filter to filter out the high-frequency signals, thus reducing the workload and errors. When performing the vibration testing, we arranged the measuring points in positions that could reflect the overall vibration characteristics of the structure. We tested each measuring point in the horizontal and directions and vertical direction in batches. Then, we selected a fixed reference point in the batch test and tested the reference point in each batch of measurement. The reference points should have good signals, and they are representative of structural vibration testing. During the data acquisition, we selected the rectangular window to maintain the original state of the impact process and transient process data. During the FFT processing data, we selected the Hann window for random signals to reduce or suppress leakage under the condition of largely reducing the height of the side lobe without widening the main lobe too much, thus effectively reducing the power leakage.
4. Vibration testing results
4.1. Vibration frequency analysis
Table 3The first-to-third-order vibration frequencies and ratios
Name of the test object | Direction | Order | Vibration frequency (Hz) | Vibration period (Sec.) | Ratio (fi/f1) |
Building A | X-direction | 1 | 3.23 | 0.310 | 1.00 |
2 | 5.28 | 0.189 | 1.63 | ||
3 | 7.62 | 0.131 | 2.36 | ||
-direction | 1 | 3.32 | 0.301 | 1.00 | |
2 | 5.38 | 0.186 | 1.62 | ||
3 | 7.43 | 0.135 | 2.24 | ||
-direction | 1 | 3.13 | 0.319 | – | |
2 | 5.87 | 0.170 | – | ||
3 | 6.84 | 0.146 | – | ||
Building B | -direction | 1 | 2.15 | 0.465 | 1.00 |
2 | 4.05 | 0.247 | 1.88 | ||
3 | 4.93 | 0.203 | 2.29 | ||
-direction | 1 | 2.49 | 0.402 | 1.00 | |
2 | 4.40 | 0.227 | 1.77 | ||
3 | 5.37 | 0.186 | 2.16 | ||
-direction | 1 | 2.39 | 0.418 | – | |
2 | 2.83 | 0.353 | – | ||
3 | 3.91 | 0.256 | – | ||
Building C | -direction | 1 | 2.74 | 0.365 | 1.00 |
2 | 4.59 | 0.218 | 1.68 | ||
3 | 6.06 | 0.165 | 2.21 | ||
-direction | 1 | 2.83 | 0.353 | 1.00 | |
2 | 4.01 | 0.249 | 1.42 | ||
3 | 6.06 | 0.165 | 2.14 | ||
-direction | 1 | 2.74 | 0.365 | – | |
2 | 4.59 | 0.218 | – | ||
3 | 6.06 | 0.165 | – |
The research results indicate that vibration frequency can provide references for the safety assessment of historical buildings [24]. In this study, we identified the dynamic parameters based on the data obtained from the test under ambient vibration and employed the signal test analysis system and modal analysis software to get vibration frequencies and mode shapes of Buildings A, B, and C in horizontal - and -directions and vertical -direction. The first-to-third-order vibration frequencies under ambient vibration are shown in Table 3. Due to the fact that the buildings mainly vibrate at low frequencies, the first-to-third vibration frequencies are typically listed.
From the perspective of dynamic characteristics of the entire test, the main vibration frequencies of three historical buildings in the -direction and -direction are within the range of 2.15 Hz-7.62 Hz, which are low. The little difference in - and -directional vibration frequencies indicates that the planar structure layout is regular, with a small difference in the transverse and longitudinal lateral stiffness of the structure. As can be seen from Table 3, the main vibration frequencies in the vertical -direction are within 2.39-3.9 1Hz, which is low. As a result, the structure is prone to vertical vibration, which is not good for earthquake resistance. The vibration frequency characteristics reflect the vertical weakness of the historical buildings with brick-wood structures. Compared with concrete floor slabs, wooden ones have lower stiffness and are weaker in connecting with vertical load-bearing members.
As can be seen from Table 3, although the vibration frequencies of three historical buildings are different in each direction, the ratio of each order frequency to the first order frequency is basically the same in each direction. The existing codes only offer the empirical formula of the basic period range, so the law can be used as the basis for the ratio of the higher order mode shape to the basic mode shape period of historic buildings with brick-wood structures, and provide references for the future formulation of codes. Besides, we can use this law to verify the correctness of the on-site testing results of dynamic characteristics.
Table 4Comparison between the calculated period based on Eq. (1) and the measured period
Name of test object | Direction | Measured period (sec.) | Constructive Eq. (1) (sec.) |
Building A | 0.310 | 0.274 | |
0.301 | 0.274 | ||
Building B | 0.465 | 0.162 | |
0.402 | 0.162 | ||
Building C | 0.365 | 0.192 | |
0.353 | 0.192 |
Moreover, the basic natural vibration period formula of multi-storey brick-concrete buildings takes H (H: building height) as the parameter. The relevant formula is shown in Eq. (1) [25]. Table 4 shows the comparison results between the calculated period based on Eq. (1) and the measured period. The results reveal a big difference between the calculated results based on the empirical formula and the measured results. The reason is that the stiffness and durability of three historical buildings decline due to deterioration and the Tangshan earthquake. The large deformation of brick-wood structures after the earthquake leads to a decrease in lateral stiffness and an increase in the vibration period:
The vibration frequency of historical buildings is subject to the age of the building, material deterioration, natural disasters, and structural layout. Fig. 4 shows the relationship between the vibration frequencies of three historical buildings and the age of the building, building height, and plane size. Building C was built about 15 years earlier than Buildings A and B, but the correlation between the first-to-third-order vibration frequencies and the age of the building is not obvious. As shown in Fig. 4(b), the vibration frequency tends to increase with the rise in building height. So, we assume that the vibration frequency of historic buildings with brick-wood structures is correlated with the building height. When the long and short axes of historic buildings suffer from the same seismic action, the direction with weak stiffness is prone to more deformation and damage. So, it is of great importance to explore the building stiffness in different directions and analyze the different directions of the resonance period [26]. Fig. 4(c) shows that the horizontal and vertical axes reveal the vibration frequencies in the and directions, respectively. The oblique line denotes the ratio of the length in the direction to the length in the direction. The plane size of Buildings A and C are similar, and the length of Building B in the direction is about 1.83 times that in the direction. However, the vibration frequencies of three buildings in the and directions are distributed along the diagonal. Therefore, we presume that the vibration period of the building in the short-axis direction is nearly equal to that in the long-axis direction, so their stiffness is considered the same. As a result, we conclude that the vibration characteristics of the building are closely related to the building shape and the configuration of the main structure.
Fig. 4Relationship between vibration frequency and the age and layout of the building: a) correlation between vibration frequency and the age of building; b) correlation between frequency and height; c) correlation between frequency and plane size
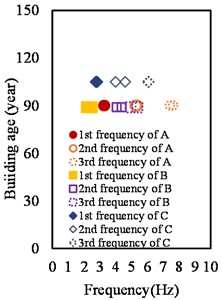
a)
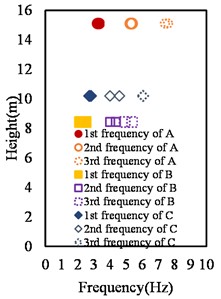
b)
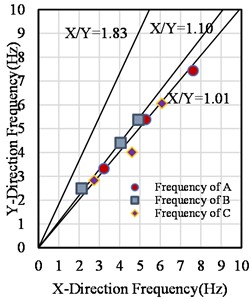
c)
4.2. Mode shape analysis
The vibration-based safety assessment is an overall assessment based on the dynamic response of the structure. There is a correspondence between the physical parameters, such as the mass and damping of the brick-wood historical buildings, and the modal parameters of the structure. The modal parameters can reflect the dynamic performance of the reaction structure. Fig. 5 shows the first-to-third-order mode shape diagrams of three historical buildings obtained from the test under ambient vibration.
According to the analysis of the first-to-third order mode shapes, the characteristics of vibration mode shapes in the and directions conform to the structural vibration characteristics within the low-frequency range, with stable vibration amplitude and good structural integrity. The first-to-third-order mode shapes mainly show translational motion. In the high-frequency stage, there may be a local vibration amplitude mutation due to the weak local connection. Additionally, the delayed torsional vibration occurs in the higher-order vibration, indicating that the plane layout of the lateral force-resisting members is reasonable, and the center of mass and the center of rigidity overlap. The vibration amplitude of the measuring points in the middle part of the long-axis direction of Building B is slightly larger than that of the measuring points on both sides, indicating that the lateral stiffness of the wall in the middle of the structure is slightly lower. The vibration amplitude of the relevant measuring points in the long-axis direction on both sides of the structure is equal, indicating that the deformation of the floor slab in the long-axis direction of the structure is weak, the internal stiffness of the floor slab is strong, and the integrity of the wall and floor slab in the long-axis direction is good. Therefore, based on the mode shape of historical buildings, we can intuitively understand the vibration characteristics of the structure and provide a scientific basis for the preservation and protection of historical buildings by long-term monitoring of potential safety hazards caused by environmental changes and material deterioration.
Fig. 5First-to-third-order mode shape diagram of historical buildings
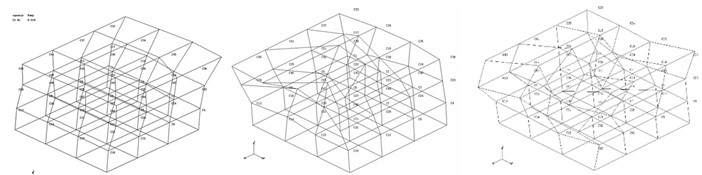
a) Building A, direction
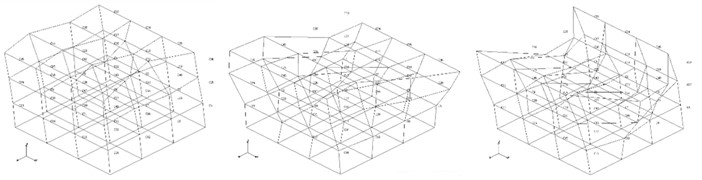
b) Building A, direction
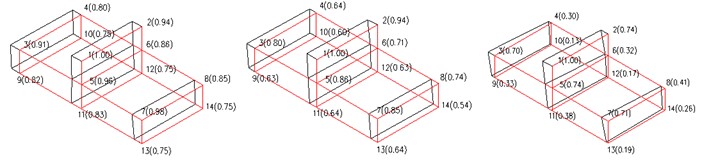
c) Building B, direction
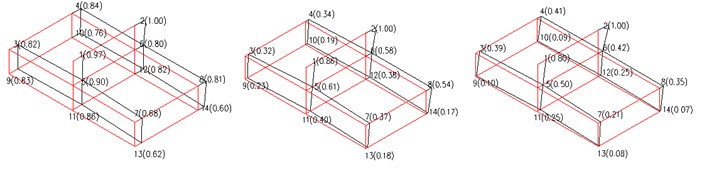
d) Building B, direction
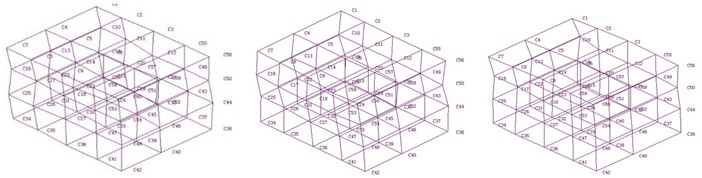
e) Building C, direction
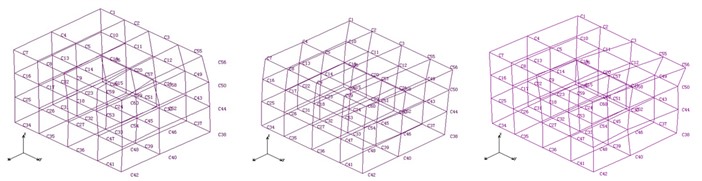
f) Building C, direction
5. Theoretical analysis results
5.1. Intrinsic value analysis based on the Stodola method
According to theories related to structural dynamics, we can use the stiffness method, flexibility method, Stodola method (Stodola -Vianello Method), Dunkerley formula, etc., to obtain natural vibration frequency. The Stodola method is a numerical method used to find the fundamental and high frequencies of the vibrating system [27].On an iterative basis, we first assume the initial mode shape and iteratively adjust it to a suitable approximation of the actual mode shape and then determine the vibration frequency based on the equations of motion. The Stodola method is used to find the natural vibration frequency and mode shape of the structure through vector iteration and performance operation. When figuring out the high frequency, it is necessary to use the orthogonality of the mode shape to modify the iteration matrix so that the calculation results converge to higher frequencies. The Stodola method is an effective method used to calculate the lowest mode shape and corresponding frequency of the structure, but its accuracy decreases gradually with the increase in the order of mode shape. Therefore, this method is characterized by controllable accuracy, strong adaptability, and accurate eigenvalues and eigenvectors at lower frequencies. In this study, we adopted the Stodola method to analyze the dynamic characteristics of the first-to-third order mode shapes of Buildings A and C. Part of Building B has three floors, but we simplified it as a two-storey building. As a result, the calculation results deviate from the measured results to some extent. For the deformation system with low linear elasticity and reliable support, it is necessary to find the flexibility matrix [] and the mass matrix [] of the system before employing the Stodola method. As the flexibility matrix is reciprocal to the stiffness matrix, we calculated the flexibility matrix using the matrix displacement method. Table 5 shows the comparison results between natural periods calculated based on the Stodola method and the measured periods. Fig. 6 shows the mode shape diagram of the intrinsic value analysis of Buildings A and C in the -direction.
Fig. 6Mode shape diagram of the intrinsic value analysis of buildings A and C
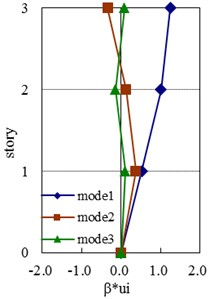
a) direction of building A
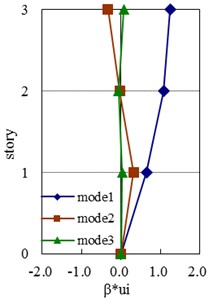
b) direction of building C
Table 5Comparison between natural periods calculated based on the Stodola method and measured periods
Architecture | Order | Period ( direction) | Period ( direction) | ||
Measured period | Calculated period | Measured period | Calculated period | ||
Building A | 1 | 0.310 | 0.260 | 0.301 | 0.256 |
Building C | 1 | 0.365 | 0.209 | 0.353 | 0.251 |
In terms of the natural period, the error between the measured and calculated results of Building A is within 20 %, while the difference between the two results of Building C is large. The overall value of the calculated nature period based on the Stodola method is lower than the vibration test results. Besides, the calculated results are close to the presumed periods shown in Table 4 based on Eq. (1). The calculation assumption does not accurately consider the material deterioration and structural damage after the earthquake, resulting in some errors. By comparing Fig. 5(a) and Fig. 6(a), we confirmed the consistency of the first-to-third-order mode shapes of Building A. Although local positions are somewhat different in Fig. 5(a) and Fig. 6(a), the overall mode shapes are almost the same. Although there are some deviations between analytical and measured results due to stiffness degradation and structural damage, the overall vibration characteristics are consistent, verifying the reliability of measured results.
5.2. Analysis of seismic performance
The seismic capacity index is an internationally used index that measures the seismic performance of engineering structures [28, 29]. Based on the Standards for Seismic Appraisal of Buildings, we evaluated the seismic performance of three brick-wood historic buildings in accordance with the comprehensive seismic capacity index of multi-storey buildings. In the first level appraisal, three buildings with historical features did not satisfy the requirements of seismic performance due to flaws in the structural system, ring beam layout, and material strength. In the second level appraisal, we calculated the comprehensive seismic capacity of three historical buildings based on the comprehensive seismic capacity index. Given that the seismic capacity index reflects the inter-storey lateral stiffness, we appraised the seismic performance of historic buildings based on the comprehensive seismic capacity index of each floor after the synergistic effect of vertical and horizontal walls and floor space. Eq. (2) shows the calculation method of the comprehensive seismic capacity index:
where, denotes the comprehensive seismic capacity index of the longitudinal or transverse wall of the -th floor; denotes the system influence coefficient; denotes the local influence coefficient; denotes the average seismic capacity index of the longitudinal or transverse wall of the -th floor.
Table 6Comprehensive seismic capacity index of three buildings with historical features
Architecture | Direction | Floor | ||
Building A | 1 | 1.36 | 0.94 | |
2 | 1.31 | 0.91 | ||
3 | 1.79 | 1.24 | ||
1 | 1.55 | 1.07 | ||
2 | 1.50 | 1.04 | ||
3 | 1.95 | 1.35 | ||
Building B | 1 | 1.53 | 1.06 | |
2 | 1.55 | 1.08 | ||
1 | 1.86 | 1.29 | ||
2 | 1.91 | 1.32 | ||
Building C | 1 | 1.56 | 1.20 | |
2 | 1.64 | 1.26 | ||
3 | 2.28 | 1.75 | ||
1 | 1.57 | 1.21 | ||
2 | 1.57 | 1.21 | ||
3 | 2.05 | 1.58 |
According to Table 6, it can be concluded that the comprehensive seismic capacity index of all floors except Building A in the -direction is greater than 1.0, indicating good seismic performance. The comprehensive seismic capacity index of the weakest floor of Building A is slightly less than 1.0. According to the first-level appraisal results, the plane layout of these three buildings with historical features is regular, with few floors and low building heights, which meet the specified requirements. Therefore, we infer that the plane layout and the height and number of floors are the main factors affecting the overall seismic performance of buildings with historical features. The conclusion is basically consistent with the vibration test results.
Meanwhile, according to the first-level appraisal results, although the strength grade of the masonry mortar of Building C' walls does not meet the relevant requirements, the comprehensive seismic capacity index of each floor of Building C is significantly higher than that of Buildings A and B. The analysis results indicate that the strength grade of masonry mortar has little influence on the overall seismic performance of the building. Moreover, based on the first-level appraisal results, the layout of the ring beam and the spacing between the seismic transverse walls of Buildings A and B do not satisfy the appraisal requirements, while the layout of the ring beam and the spacing between the seismic transverse walls of Building C meet the appraisal requirements. Therefore, we infer that the overall connection structure and the spacing between the seismic transverse walls have great impacts on the overall seismic performance of the building.
5.3. Comparison of experimental results
The seismic performance analysis reveals that the main factors affecting the overall seismic performance of historical buildings are the height and number of floors, the plane layout, the spacing between the seismic transverse walls in the structural system, and the overall connection structure, while the strength grade of masonry mortar has little effects on the overall seismic performance of historical buildings. As shown in Fig. 4, the vibration characteristics of the structure are correlated with the height and layout of the building, the structural characteristics reflected by vibration measurement and seismic performance analysis results are relatively consistent. Besides, the comprehensive seismic capacity index of the -direction floor of the structure is lower than that of the -direction, indicating that the -direction is the weak direction of the structure, which is consistent with the measured results as shown in Table 4 and verifies the reliability of the vibration test results.
6. Conclusions
In this study, we explored three historical buildings with brick-wood structures in Tianjin and drew the following conclusions based on the testing under ambient vibration, dynamic characteristics analysis, comparison of the results of intrinsic value analysis, and calculation of seismic performance.
1) The validity of testing under ambient vibration has been verified. Based on the analysis of vibration frequencies and mode shapes, we have accurately figured out the dynamic characteristics of historical buildings with brick-wood structures. The vibration frequencies of brick-wood buildings with historical features are generally low. Through mode shape analysis, it is concluded that the integrity of the structure is good without obvious structural defects. Vibration characteristics are bound up with the shape of the building and the configuration of the main structure. Meanwhile, the structural stiffness is subject to the age of the building, material deterioration, natural disasters, and structural layout.
2) There are some errors between the intrinsic value analysis results and vibration test results. This may occur due to the long history of historical buildings with brick-wood structures, which leads to a decline in structural stiffness and structural damage. The overall mode shapes of the two are consistent, and the reliability of the measured results has been verified. We should take full consideration of stiffness reduction caused by the deterioration and damage of historical buildings in future simulation analysis.
3) The seismic performance analysis reveals that the plane layout and the number of floors of the historic building are the main factors affecting the overall seismic performance. Besides, the connection structure and the spacing between the seismic transverse walls have greater impacts on the overall seismic performance of the building. Additionally, the characteristics of the comprehensive seismic capacity index are consistent with the results of the dynamic characteristic analysis.
In this study, we explored the dynamic characteristics, performed the intrinsic value analysis and seismic performance analysis of three historical buildings with brick-wood structures, and made some achievements in theory and practice. However, due to the complexity of historical buildings, it is necessary to accumulate research data to perform in-depth research on more issues. Moreover, the dynamic performance of the structure changes over time, and the monitoring of its changes can indirectly reflect the health status of the structure. The periodic diagnosis based on vibration testing can provide reliable references for future health detection and condition evaluation of historical buildings.
References
-
B. Wu, P. Liu, W. Huang, and G. Meng, “Safety assessment of ancient buildings under adjacent subway blasting construction based on the optimized fuzzy optimal method,” Shock and Vibration, Vol. 2021, pp. 1–14, Nov. 2021, https://doi.org/10.1155/2021/2573201
-
A. H. A. Dehwah, A. Haredy, and M. Krarti, “Retrofit analysis of historical buildings to net-zero energy: Case study of the Ain village, Saudi Arabia,” Energy and Buildings, Vol. 258, p. 111826, Mar. 2022, https://doi.org/10.1016/j.enbuild.2021.111826
-
A. Barontini and P. B. Lourenço, “Seismic safety assessment of mixed timber-masonry historical building: an example in Lima, Peru,” Journal of Earthquake Engineering, Vol. 25, No. 5, pp. 872–891, Apr. 2021, https://doi.org/10.1080/13632469.2018.1540368
-
W. Ji and J. Li, “A comparative study on the renewal practice modes of historic buildings in China and Japan-Taking Tianjin, Kobe, and Maizuru as examples,” in IOP Conference Series: Earth and Environmental Science, Vol. 825, No. 1, p. 012031, Jul. 2021, https://doi.org/10.1088/1755-1315/825/1/012031
-
Tianjin Administration of Land Resources and Housing, “The protective utilization and technological innovation of Tianjin historical architectures,” City and House, No. 12, pp. 41–46, 2015.
-
Y. Cao et al., “Seismic performance evaluation of typical modern brick-wood structures in Tian-jin based on safety assessment,” China Earthquake Engineering Journal, Vol. 41, No. 4, pp. 880–886, 2019, https://doi.org/10.3969/j.issn.1000-0844.2019.04.880
-
A. Alekseytsev, M. Botagovsky, and N. Kurchenko, “Cost minimization for safety enhancing of timber beam structures in historical buildings,” E3S Web of Conferences, Vol. 97, p. 03002, 2019, https://doi.org/10.1051/e3sconf/20199703002
-
J. Radnić, D. Matešan, and A. Abaza, “Restoration and strengthening of historical buildings: The example of Minceta Fortress in Dubrovnik,” Advances in Civil Engineering, Vol. 2020, pp. 1–17, Sep. 2020, https://doi.org/10.1155/2020/8854397
-
G. Q. Zhou et al., “Appraisal and reinforcement for a modern historical building,” Building Structure, Vol. 50, No. 6, pp. 25–28, 2020, https://doi.org/10.19701/j.jzjg.2020.06.005
-
W. Wang, B. C. Hua, and J. Yao, “Research and exploration no the seismic performance improvement of historical building,” Building Structure, Vol. 52, No. 3, pp. 67–72, 2022, https://doi.org/10.19701/j.jzjg.ls201860
-
W. He, H. Luo, W. Chang, H. Xu, W. Liu, and Q. Zhang, “Experiment investigation and in situ test of hybrid vibration bearing system applied to overtrack historical buildings,” Structural Control and Health Monitoring, Vol. 29, No. 4, Apr. 2022, https://doi.org/10.1002/stc.2921
-
G. Boscato, A. Dal Cin, and S. Russo, “Collapse mechanisms due to earthquake in the structural typologies of historic constructions: the case of Mirandola,” Key Engineering Materials, Vol. 624, pp. 59–65, Sep. 2014, https://doi.org/10.4028/www.scientific.net/kem.624.59
-
N. R. Tibon and R. M. Suiza, “Quantification of seismic exposure and vulnerability of historic buildings in Metro Manila,” International Journal of Disaster Resilience in the Built Environment, Vol. 9, No. 3, pp. 249–257, Jun. 2018, https://doi.org/10.1108/ijdrbe-01-2017-0010
-
V. Smirnov, “Vibration protection of historical buildings located near the lines of urban rail transport,” Materials Science Forum, Vol. 945, pp. 318–324, Feb. 2019, https://doi.org/10.4028/www.scientific.net/msf.945.318
-
S. L. Lu, “Study on dynamic characteristics of historic buildings,” Tianjin University, Tianjin, 2014.
-
S. H. Dong, “Study on the seismic performance of masonry-timber historic buildings,” Tianjin University, Tianjin, 2012.
-
E. N. Farsangi et al., “Ambient vibration testings and field investigations of two historical buildings in Europe,” Structural Durability and Health Monitoring, Vol. 14, No. 4, pp. 283–301, 2020, https://doi.org/10.32604/sdhm.2020.010564
-
E. Nochebuena-Mora, N. Mendes, P. B. Lourenço, and J. A. Covas, “Vibration control systems: A review of their application to historical unreinforced masonry buildings,” Journal of Building Engineering, Vol. 44, p. 103333, Dec. 2021, https://doi.org/10.1016/j.jobe.2021.103333
-
N. Fiorini, G. Acunzo, D. Spina, and G. Falzone, “Structural Health Assessment of Historical Buildings via Ambient Vibrations: The SMAV Methodology for “Palazzo delle Laudi” at Sansepolcro (AR),” International Journal of Architectural Heritage, Vol. 15, No. 4, pp. 593–607, Apr. 2021, https://doi.org/10.1080/15583058.2019.1636330
-
C. Y. Qian et al., “Influence study on subway construction and operation vibration on cultural relics buildings,” Building Structure, Vol. 45, No. 19, pp. 87–91, 2015, https://doi.org/10.19701/j.jzjg.2015.19.018
-
Y. Zhou, X. Bai, S. Wang, K. Zhao, H. Su, and H. Wang, “Structural vibration analysis of historical drum tower structure caused by underground train running,” Frontiers in Earth Science, Vol. 9, p. 808123, Jan. 2022, https://doi.org/10.3389/feart.2021.808123
-
A. Shishegaran, M. Moradi, M. A. Naghsh, B. Karami, and A. Shishegaran, “Prediction of the load-carrying capacity of reinforced concrete connections under post-earthquake fire,” Journal of Zhejiang University-SCIENCE A, Vol. 22, No. 6, pp. 441–466, Jun. 2021, https://doi.org/10.1631/jzus.a2000268
-
A. Fathi, A. Sadeghi, M. R. Emami Azadi, and N. Hoveidaie, “Assessing seismic behavior of a masonry historic building considering soil-foundation-structure interaction (Case Study of Arge-Tabriz),” International Journal of Architectural Heritage, Vol. 14, No. 6, pp. 795–810, Jul. 2020, https://doi.org/10.1080/15583058.2019.1568615
-
F. Ubertini, G. Comanducci, N. Cavalagli, A. Laura Pisello, A. Luigi Materazzi, and F. Cotana, “Environmental effects on natural frequencies of the San Pietro bell tower in Perugia, Italy, and their removal for structural performance assessment,” Mechanical Systems and Signal Processing, Vol. 82, pp. 307–322, Jan. 2017, https://doi.org/10.1016/j.ymssp.2016.05.025
-
X. Q. Yao et al., “Elasto-plastic finite element analysis of typical brick-wood rural buildings under ground motions,” China Earthquake Engineering Journal, Vol. 40, No. 1, pp. 32–40, 2018, https://doi.org/10.3969/j.issn.1000-0844.2018.01.0.32
-
Narenmandula et al., “Regional differences and dynamic characteristics of RC buildings based on microtremor measurement,” Journal of Vibration and Shock, Vol. 35, No. 4, pp. 22–27, 2016, https://doi.org/10.13465/j.cnki.jvs.2016.04.004
-
C. Q. Tao et al., “Study of structural dynamic characteristics based on Stodola method and Gauss-Jordan method,” Sichuan Building Science, Vol. 37, No. 1, pp. 175–177, 2011.
-
K. Fujii, “Pushover-based seismic capacity evaluation of Uto city hall damaged by the 2016 Kumamoto earthquake,” Buildings, Vol. 9, No. 6, p. 140, Jun. 2019, https://doi.org/10.3390/buildings9060140
-
Z. Y. Wang et al., “Comprehensive evaluation of seismic performance of farmhouse,” Building Structure, Vol. 52, No. 17, pp. 39–44, 2022, https://doi.org/10.19701/j.jzjg.20201335
About this article
This research was supported by Scientific Research Project of Tianjin Education Committee (2022KJ044).
The datasets generated during and/or analyzed during the current study are available from the corresponding author on reasonable request.
Conceptualization, Liang Li and Jianjiang Yang; methodology, Jianjiang Yang; software, Ping Liu.; validation, Liang Li and Jianjiang Yang; formal analysis, Liang Li; investigation, Jianjiang Yang; resources, Jianhua Fu; data curation, Jianjiang Yang; writing-original draft preparation, Liang Li; writing-review and editing, Liang Li; visualization, Liang Li. All authors have read and agreed to the published version of the manuscript.
The authors declare that they have no conflict of interest.